Relativistic and Ultrashort Quantum Dynamics
Since the invention of laser light amplification with chirped pulses, extremely short and strong laser fields have been generated with ever-increasing intensities. While current lasers reach up to 1023 W/cm2, the European Extreme-light-infrastructure (ELI) project aims at much higher fantastic intensities, which will allow testing QED in nonlinear and non-perturbative regimes. Strong fields at short wavelengths are also accessible, among them a free-electron laser for a strong XUV radiation (FLASH facility) at DESY (Hamburg), x-ray free-electron lasers (XFEL) at DESY, and LCLS (Linac Coherent Light Source) in SLAC (Stanford, USA). With these large-scale facilities, nonlinear x-ray physics can be probed. Many exciting projects have been put forward using these machines, to mention only a few of them: the LUXE experiment (Laser Und XFEL Experiment) is in planning at DESY, using the electron beam of the European XFEL, and aimed at non-perturbative QED at the strong-field frontier. The latter also will be tested in the planned FACET-II facility at SLAC via beam-beam collisions. Moreover, experimentalists can produce well-controlled ultrashort and tailored laser pulses and carry out precise measurements of the electron and ion momenta in coincidence during strong-field ionization of atoms and molecules via a so-called reaction microscope. These achievements offer new methods for monitoring the bound electron dynamics with Angstrom spatial and attosecond temporal resolution, thus paving the way for efficient control of atomic and molecular processes on the nanoscale. Thus, there is a bright outlook for the investigation of strong laser radiation interacting with matter, and strong-field physics.
We investigate the interaction of strong laser radiation with matter. The systems under consideration range from free electrons, electron beams, single atoms/ions, few-atom ensembles, thin matter layers, and plasmas up to vacuum with quantum fluctuations. At the center of interest are the relativistic regimes of interaction. In particular, our attention is focused on nonlinear ionization dynamics in strong fields, as well as on radiative and nonlinear QED effects in strong fields. Our recent results include an understanding of the role of sub-barrier as well as nondipole dynamics for shaping subtle features of the photoelectron momentum distribution in tunneling ionization, which shed light on the elusive notion of the tunneling time, nondipole time delay for atoms and molecules, as well as uncovers the nondipole signatures of the sub-barrier dynamics. In the direction of nonlinear QED during ultrastrong laser-electron beam interaction, we have recently put forward new methods for obtaining highly polarized electron and positron beams in femtosecond time scale using ultrastrong laser fields, as well as demonstrated efficient ways for transferring polarization from relativistic electrons to high-energy gamma-photons, and elucidated the essential role of the electron anomalous magnetic moment in these processes. We elaborated on new diagnostic methods of plasma employing the polarization properties of the emitted gamma photons and ultrarelativistic electrons from plasma.
Selected results: Strong-field ionization
Signatures of under-the-barrier dynamics in tunneling ionization
In a strong laser field, ionization of an atom takes place via tunneling of the electron from the atomic bound state into the continuum through the potential barrier formed by the atomic potential and the laser field. Although the under-the-barrier dynamics is a small part of the whole laser-electron interaction in this process, it imprints its gentle signatures in the photoelectron momentum distribution at the detector. A high resolution of momentum detection, better than 0.01 atomic units, is required to observe these signatures. One of these signatures is the time delay in the attoclock, which has been measured in a mixture of gases in [1]. In the latter, the systematic experimental errors have been canceled by measuring the difference in the time delay for two gases. We have given successful theoretical explanations of these experimental results via the Wigner time delay during sub-barrier dynamics, see Fig. 1. While there are debates on how to interpret the attoclock time delay, we put forward a simple straightforward interpretation in [2]. The time delay is equivalent to a shift in the momentum distribution of the attoclock, we have shown that the latter emerges when the interference of the direct ionization path with the under-the-barrier recolliding one is accounted for, see Fig 2.
In our recent work [3] we have emphasized that two faces of the concept of the tunneling time delay should be clearly distinguished to resolve the debate on the attoclock time delay. While the asymptotic time delay (ATD) is read out from the asymptotic photoelectron momentum distribution, see Fig.3, the near-exit time delay (ETD) is considered theoretically, observable in a Gedanken experiment with a so-called virtual detector near the tunnel exit (ETD). The ATD and ETD characterise the tunneling dynamics from different perspectives: While ATD is related to the attoclock protocol, ETD describes how the classical trajectory emerges from the quantum dynamics of the laser-driven atomic electron. In [4] we showed that a positive ETD exists as a matter of principle and arises due to the sub-barrier interference of the reflected and transmitted components of the tunneling electronic wave packet. In [3] we reconcile conflicting approaches for the tunneling time delay in strong-field ionization. The peak of the tunneling wave packet emerging from the barrier around the tunnel exit significantly deviates from the most probable classical backpropagated trajectory, featuring a positive ETD. It however asymptotically merges with the backpropagated trajectory, which itself shows negative ATD, originating from the interference of the direct and recolliding sub-barrier paths. Finally, in explaining the absence of tunneling times from the other methods, we have clarified that there are indeed no conflicts among the various approaches.
[1] N. Camus, et al. Phys. Rev. Lett. 119, 023201 (2017).
[2] M. Klaiber, K. Z. Hatsagortsyan, and C. H. Keitel, Phys. Rev. Lett. 120, 013201 (2018).
Nondipole effects in strong-field ionization
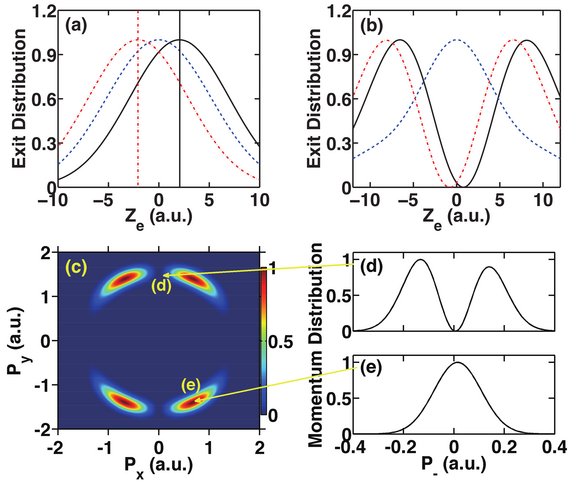
In the strong-field ionization problem, the nondipole effects are exhibited in the continuum dynamics of the ionized electron, as well as at the ionization during sub-barrier motion. We predicted in [1] a signature of the under-the-barrier dynamics due to the nondipole effect of the laser magnetic field. The peak of the electron momentum distribution along the laser propagation direction is shifted forward at the tunnel exit during the under-the-barrier wave packet formation. It has a consequence on the photon momentum partition between the ion and the electron in the ionization process. Our fully relativistic prediction has been confirmed by an external group in ultra-high precision measurement in [2].
In our recent study [3] we have examined the role of the Coulomb field of the atomic core for the nondipole under-the-barrier and revealed its counterintuitive nature. Despite its attractive nature, the sub-barrier Coulomb field increases the photoelectron nondipole momentum shift along the laser propagation direction, involving a strong dependence on the laser field. The scaling of the effect concerning the principal quantum number and angular momentum of the bound state is found. We demonstrate that the signature of Coulomb-induced sub-barrier effects can be identified in the asymptotic photoelectron momentum distribution via a comparative study of the field-dependent longitudinal momentum shift for different atomic species with state-of-the-art experimental techniques of mid-infrared lasers.
The nondipole strong-field ionization time delay in a molecule due to the finiteness of the light speed is investigated in [4]. The nondipole ionization delay manifests itself in the longitudinal momentum distribution, featuring double-slit interference, which efficiently imprints the molecule structure and laser parameters. We found that the delay depends essentially on the tunneling exit distribution, rather than the molecular bond length. For rare gas dimers, the bright fringe in the polarization plane has a single longitudinal peak, while the dark fringe has double longitudinal peaks, see Fig.1. We show also that the tunneling exit distribution of an atom with a nonzero angular momentum also features two tunneling exits, which yields a nontrivial time delay as a consequence of destructive double-slit interference.
[1] M. Klaiber, et al. , Phys. Rev. Lett. 110, 153004 (2013).
[2] A. Hartung, et al. Nat. Phys. 15, 1222 (2019).
[3] P. He, M. Klaiber, K. Z. Hatsagortsyan, and C. H. Keitel, Phys. Rev. A 105, L031102 (2022).
[4] P. He, K. Z. Hatsagortsyan, and C. H. Keitel, Phys. Rev. Lett. 128, 183201 (2022).
Interplay between Coulomb-focusing and nondipole effects in strong-field ionization with elliptical polarization
In collaboration with an experimental group of ETH, Zürich, we studied strong-field ionization and rescattering in the nondipole regime with elliptically polarized mid-IR laser pulses. An unexpected sharp ridge structure in the polarization plane in photoelectron momentum distribution (PMD) has been discovered [1], see Fig. Within a certain range of ellipticity, the electrons in this ridge are clearly separated from the two lobes that commonly appear in the PMD with elliptically polarized laser fields. In contrast to the well-known lobes of direct electrons, the sharp ridge is created by Coulomb focusing of the softly recolliding electrons. It appeared that this thin line-shaped ridge structure for low-energy photoelectrons is correlated with the ellipticity-dependent asymmetry of the PMD along the beam propagation direction. The peak of the projection of the PMD onto the beam propagation axis is shifted from negative to positive values when the sharp ridge fades away with increasing ellipticity [2]. Our theoretical analysis [3] showed that the underlying physics is based on the interplay between the lateral drift of the ionized electron, the laser magnetic field induced drift in the laser propagation direction, and Coulomb focusing. The ellipticity-dependent 3D PMDs give access to different ionization and recollision dynamics with appropriate filters in the momentum space. For example, we can extract information about the spread of the initial wave packet and the Coulomb momentum transfer of the rescattering electrons.
Within the same collaboration, we have investigated also the influence of the laser magnetic-field component onto the holographic interference pattern [4] (the interferences between electron pathways that are driven directly to the detector and those that rescatter significantly with the parent ion lead to holography-type interference). We provided explanations for the experimentally demonstrated asymmetry in the holographic interference pattern and for the variation of the topology of the holography-type interference pattern along the laser-field direction.
[1] J. Mauer, et al. Phys. Rev. A 97, 013404 (2018).
[2] J. Daněk, et al. J. Phys B 51, 114001 (2018).
[3] J. Daněk, K. Z. Hatsagortsyan, and C. H. Keitel, Phys. Rev. A 97, 063409, 063410 (2018).
Coulomb effects in strong-field processes
The Coulomb field of the atomic core plays a significant role in strong-field ionization. For a long time, it has been known that significant Coulomb effects arise at recollisions. While hard recollisions induce well-known processes of above-threshold ionization, high-order harmonic generation, and nonsequential double ionization, the soft recollisions bring about Coulomb focusing effect, which is responsible for the so-called, low-energy structures (LESs) in the photoelectron energy distribution at above threshold-ionization (ATI) in mid-infrared laser fields. We have explained the origin of the low-energy structure [1], which arises due to Coulomb focusing because of multiple forward scattering of the ionized electron by the parent ion, see Fig.1 A surprising fact was that the high-order scattering events have a nonperturbative comparable contribution to the total Coulomb focusing [2], and persist up to high ellipticity values of the driving laser field [3].
Recently, another surprising Coulomb field effect has been identified by ab initio numerical solution of time-dependent Schrödinger equation (TDSE): several orders enhancement of photoelectron spectra in the upper energy range of the direct electrons, i.e., at 2Up, twice the electron ponderomotive energy, has been observed. We have demonstrated [4] that the enhancement is of a classical origin. It is due to the longitudinal nonuniform Coulomb momentum transfer with respect to the ionization phase, which allows for the electrons tunneled not far from the peak of the laser field to accumulate at high energies, Fig.2. Moreover, our analysis reveals specific features of the angular distribution of high-energy direct electrons, which can be employed for molecular imaging.
The role of the Coulomb field for attoclock we have analyzed in [5] and showed that it is especially increased in the nonadiabatic regime. The latter is due to the closer tunnel exit coordinate to the atomic core in the nonadiabatic regime when the electron gains energy during tunneling.
[1] C. Liu and K. Z. Hatsagortsyan, Phys. Rev. Lett. 105, 113003 (2010).
[2] C. Liu and K. Z. Hatsagortsyan, J. Phys. B 44, 095402 (2011).
[3] C. Liu and K. Z. Hatsagortsyan, Phys. Rev. A 85, 023413 (2012).
[4] P. He, et al. , Phys. Rev. A 98, 053428 (2018).
[5] M. Klaiber, K. Z. Hatsagortsyan, and C. H. Keitel, Phys. Rev. Lett.114, 083001 (2015).
Spin dynamics in relativistic ionization
Spin effects arise during the relativistic tunneling ionization process [1]. We have investigated the spin-resolved ionization dynamics employing the relativistic Coulomb corrected dressed strong field approximation [2], and taking into account the laser field-driven electron spin dynamics in the bound state. Even if an electron is very tightly bound to an ionic core, it may still be crucially affected by a laser field of moderate intensity. Spin effects in the tunneling regime of ionization are built up in three steps: spin precession in the bound state, spin rotation during tunneling, and spin precession during the electron motion in the continuum, see Fig. The magnitude and scaling of the spin-flip and spin asymmetry effects at ionization are reduced when the electron spin dynamics in the bound state is taken into account. However, with super-strong laser fields, a large spin-flip effect is measurable when employing highly charged ions, initially polarized along the laser propagation direction. The anticipated spin-flip effect is expected to be measurable with modern laser techniques combined with an ion storage facility.
Relativistic high-order harmonic generation (HHG)
We have investigated the ways for extension of the ionization-recollision dynamics to the relativistic domain as a pathway to radiation sources in a hard x-ray domain via HHG. To this purpose, we consider different setups for the suppression of the magnetically induced drift in the relativistic regimes of HHG.
For example, the XUV or x-ray assistance can be employed to overcome the relativistic drift motion. Another possibility for relativistic HHG can be achieved by employing strong laser pulses tailored as an attosecond pulse train. The temporal tailoring of the laser pulse is intended to concentrate the ionizing and accelerating laser forces in short time intervals within the laser period, maintaining the average intensity of the pulse constant. This is because in the tailored laser pulse, fragments are avoided in the electron trajectory, in contrast to the sinusoidal laser pulse, where the electron acceleration is compensated by deceleration without a net energy gain by the electron, while the electron nevertheless continues to drift in the laser propagation direction. What appears particularly promising for the suppression of the relativistic drift is the use of an HHG scheme with counter-propagating attosecond pulse trains where a special method for phase-matching has been developed. The review of different schemes is given in [1].
Selected results: nonlinear QED
Laser-induced polarization of electron and positron beams
Relativistic polarized electron and positron beams are fundamental experimental tools to test symmetry properties in physics. We showed a way to polarize electron and positron beams with currently available realistic laser fields [1]. The nonlinear interaction of electrons with an elliptically polarized laser field resulted in the splitting of the beam with respect to polarization due to the spin dependence of radiation reaction, see Fig. 1. The asymmetry of the photon emission probabilities w.r.t. spin is particularly significant in high-energy photon spectra and can be employed for the development of a polarimetry method [2]. Furthermore, in strong external fields, the electron-positron pair production probabilities possess much higher asymmetry w.r.t. the spin of the created particles than the radiation. The latter property was used for the generation of highly polarized positrons in a two-color laser field possessing a high degree of asymmetry [3], see Fig. 2, and in a laser field with a small ellipticity [4].
Generation of circularly polarized (CP) and linearly polarized (LP) γ-rays via the single-shot interaction of an ultraintense laser pulse with a spin-polarized counterpropagating ultrarelativistic electron beam was demonstrated in nonlinear Compton scattering in the quantum radiation-dominated regime [5]. We showed efficient ways for the transfer the electron polarization to high-energy photon polarization. Resolving for the intermediate gamma-photon polarization could be important even without polarization measurement of outgoing particles. This was in particular shown in the multiphoton Breit–Wheeler pair production process during the interaction of an ultrarelativistic electron beam with a counterpropagating elliptically polarized laser pulse~[6].
Recently, we have analyzed the role of the anomalous magnetic moment and no-photon-emission spin dynamics, i.e., effects which both are consequences of QED radiative corrections, for electron polarization in the radiation-dominated regime with multiple photon emissions [7]. We have shown that exclusively due to these effects helicity transfer is possible from circularly polarized laser photons to electrons in an ultrastrong field regime, which challenged the belief that circularly polarized laser beams cannot induce high longitudinal polarization. The helicity transfer happens via an intermediate step of the electron radiative transverse polarization, phase-matched with the driving field, followed up by spin rotation into the longitudinal direction as induced by the anomalous magnetic moment of the electron, see Fig.3. This effect is detectable with currently achievable laser facilities, evidencing the role of the leading QED vertex correction to the electron anomalous magnetic moment in the polarization dynamics in ultrastrong laser fields
[1] Y. Li, et al., Phys. Rev. Lett. 122, 154801 (2019).
[2] Y. Li, et al., Phys. Rev. Applied 12, 014047 (2019).
[3] Y. Chen, et al., Phys. Rev. Lett. 123, 174801 (2019).
[4] F. Wan, et al., Phys. Lett. B 800, 135120 (2019).
[5] Y. Li, et al. Phys. Rev. Lett. 124, 014801 (2020).
[6] F. Wan, Y. Wang, R. Guo, Y. Chen, R. Shaisultanov, Z. Xu, K. Z. Hatsagortsyan, C. H. Keitel, and J. Li, Phys. Rev. Research 2, 032049(R) (2020);Y. Dai, B. Shen, J. Li, R. Shaisultanov, K. Z. Hatsagortsyan, C. H. Keitel, and Y. Chen, Mat. Rad. Extrem. 7, 014401 (2021);K. Xue, R.-T. Guo, F. Wan, R. Shaisultanov, Y.-Y. Chen, Z.-F. Xu, X.-G. Ren, K. Z. Hatsagortsyan, C. H. Keitel, J.-X. Li, Fundamental Research (2022).
[7] Y.-F. Li, Y.-Y. Chen, K. Z. Hatsagortsyan, and C. H. Keitel, Phys. Rev. Lett. 128, 174801 (2022).
Plasma imaging via polarization properties of ejected particles
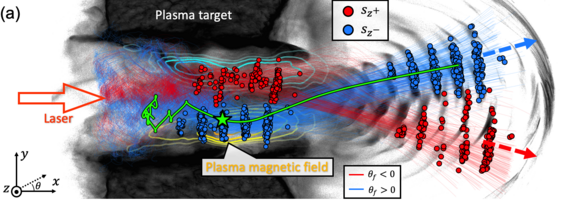
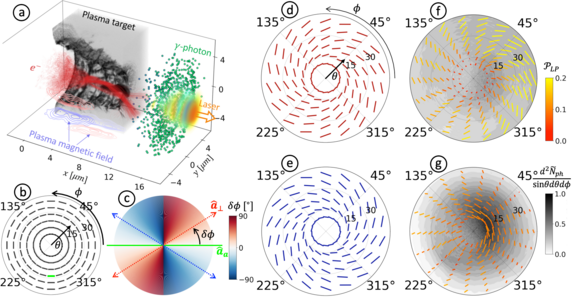
Understanding and interpreting the dynamics of ultrarelativistic plasma is a challenge, which calls for the development of methods for in situ probing the plasma dynamical characteristics. The ejected electron spin provides a new degree of freedom to extract information on the structure and magnitude of different components of the transient plasma fields. We have investigated the interaction of an ultrastrong short laser pulse with nonprepolarized near-critical density plasma in an ultrarelativistic regime, with an emphasis on the radiative spin polarization of ejected electrons [1]. Our particle-in-cell simulations show explicit correlations between the angle-resolved electron polarization and the structure and properties of the transient quasistatic plasma magnetic field, see Fig.1. While the magnitude of the spin signal is the indicator of the magnetic field strength created by the longitudinal electron current, the asymmetry of electron polarization is found to gauge the island-like magnetic distribution which emerges due to the transverse current induced by the laser wavefront. Our results open a new avenue for electron spin-based plasma diagnostics in extreme conditions, which are prevalent in astrophysical environments and are expected in near future laser facilities.
We also put forward a new method, harnessing polarization properties of γ -photons emitted from a non-prepolarized plasma irradiated by a circularly polarized pulse [2]. We show that the angular pattern of γ -photon linear polarization is explicitly correlated with the dynamics of the radiating electrons, which provides information on the laser-plasma interaction regime, see Fig.2. Furthermore, with the γ -photon circular polarization originating from the electron radiative spin flips, the plasma susceptibility to quantum electrodynamical processes is gauged. Our diagnostic scheme via the emitted γ -photon polarization is mainly applied in the laser-driven ultrarelativistic overdense plasma, which cannot be measured by the conventional optical probes. In addition, since the ultrarelativistic plasma is generally associated with sufficiently strong fields, the requirement of the ion energy on the proton radiography is not feasible either. Therefore, the advantage of the diagnostic based on γ-photon polarization is its applicability in the energetic and overdense plasma. This diagnostic tool may appear beneficial for a better understanding of phenomena in broad high-intensity interaction scenarios including ion acceleration, direct laser acceleration, high-harmonic generation, brilliant photon emission, ultradense nanopinches, and e−e+ pair plasma cascades.
[1] Z. Gong, K. Z. Hatsagortsyan, and C. H. Keitel, Phys. Rev. Lett. 127, 165002 (2021).
[2] Z. Gong, K. Z. Hatsagortsyan, and C. H. Keitel, Phys. Rev. Res. 4, L022024 (2022).
Gamma-rays with ultrastrong laser and electron beam interaction
The advent of petawatt-class lasers enables GeV electron beams in strong electromagnetic fields to be studied experimentally using all-optical systems. We have studied several promising configurations that explore previously untested regimes. A setup of a unique x-ray source is put forward employing a relativistic electron beam interacting with two counterpropagating laser pulses in the nonlinear few-photon regime [1,2]. The electron beam co-propagate along the stronger laser wave, while the counterpropagating laser beam is comparatevely weak. The electron motion features two typical frequencies separated by orders of magnitude because of the Doppler effect. The electron absorbs several photons in both frequencies in the considered regime when emitting an x-ray photon. As a result, in the emission spectrum the Doppler-shifted high frequency peak is accompanied with satellites. While the gross features of the spectrum (the spectral envelope) are determined by the counterpropagating laser beam, the subtle features are governed by the second copropagating laser beam. Accordingly, the bandwidth of satellites scales with the smaller characteristic frequency, allowing for bright ultranarrow x-rays. In contrast to Compton scattering sources, the envisaged x-ray source exhibits an extremely narrow relative bandwidth of the order of 10−4 and the brilliance of the x rays can be an order of magnitude higher than that of a state-of-the-art Compton source. By tuning the laser intensities and the electron energy, one can realize either a single peak or a comblike x-ray source in the range of several hundreds of eV to tens of keV. The laser intensity and the electron energy in the suggested setup are rather moderate, rendering this scheme compact and tabletop size, as opposed to x-ray free-electron laser and synchrotron infrastructures. This radiation source is attractive for several applications, for instance, for resonant excitation spectroscopy of highly charged ions, for seeding of x-ray free-electron laser, or for extension of plasma diagnostics from the optical and UV range to the hard x-ray domain. Finally, replacing the laser fields with strong THz ones, the
presented idea can be extended to a hard x-ray frequency comb for ultrahigh precision metrology.
The local constant field approximation (LCFA) is widely used in the study of QED effects in laser-matter interactions, with the justification that the classical strong-field parameter of the impinging laser beam is large. We demonstrated the failure of this conjecture for an electron interacting with strong counterpropagating laser waves due to the emergence of an additional small time scale in the electron dynamics. Moreover, we identify a class of anomalous LCFA violation in which electrons turn sharply and leave the radiation formation zone much earlier than in the LCFA estimation, see Fig.14. In contrast to previous observations of LCFA violation in a single laser beam, resulting in new low-harmonic peaks in the spectrum, here deviations from LCFA results are seen across the whole spectrum. A similar phenomenon is also demonstrated for an electron colliding with an ultrashort laser pulse. These results indicate the necessity of amending laser-plasma kinetic simulations in multiple beam laser configurations.
The feasibility of the generation of bright ultrashort gamma-ray pulses we have demonstrated in the interaction of a relativistic electron bunch with a counterpropagating tightly focused superstrong laser beam in the radiation-dominated regime [3]. Ultrashort gamma-ray bursts of hundreds of attoseconds and of dozens of megaelectronvolt photon energies in the near-backward direction of the initial electron motion can be generated, which is due to the tightly focused laser field structure and the radiation reaction. Interesting is that the duration of the gamma-ray burst is independent of the duration of the electron bunch and of the laser pulse.
Radiation of an electron bunch in a superstrong focused ultrashort laser pulse of variable duration can provide signatures of quantum radiation reaction. These are visible in the qualitative behavior of both the angular spread and the spectral bandwidth of the radiation spectra. The signatures are robust with respect to the variation of the electron and laser-beam parameters in a large range. In the same setup, we have identified a CEP effect specific to the ultrarelativistic regime [4]. When the electron beam counterpropagates with the laser pulse, pronounced high-energy x-ray double peaks emerge near the backward direction relative to the initial electron motion, Fig.3. This is achieved in the relativistic interaction domain, where both the electron energy is required to be lower than for the electron reflection condition at the laser peak and the stochasticity effects in the photon emission need to be weak. The asymmetry parameter of the double peaks in the angular radiation distribution is shown to serve as a sensitive measure for the CEP of up to 10-cycle long laser pulses and can be applied for the characterization of extremely strong laser pulses in present and near future laser facilities.
Gamma-ray beams with a large angular momentum may affect astrophysical phenomena, which calls for appropriate earth-based experimental investigations. For this purpose, we have investigated [5] the generation of well-collimated gamma-ray beams with a very large orbital angular momentum using nonlinear Compton scattering of a strong laser pulse of twisted photons at ultrarelativistic electrons. Angular momentum conservation among absorbed laser photons, quantum radiation, and electrons is numerically demonstrated in the quantum radiation-dominated regime. We point out that the angular momentum of the absorbed laser photons is not solely transferred to the emitted gamma photons, but due to radiation reaction shared between the gamma photons and interacting electrons. The accompanying process of electron-positron pair production is furthermore shown to enhance the orbital angular momentum gained by the gamma-ray beam.
[2] Q. Z. Lv, E. Raicher, C. H. Keitel, and K. Z. Hatsagortsyan, Physical Review Res. 3, 013214 (2021).
[3] J. Li, et al., Phys. Rev. Lett. 115, 204801 (2015).
High-energy electron-positron collider
For the high energy domain of laser physics, we have proposed the concept of a laser-driven high-energy electron-positron collider which employs a bunch of positronium atoms [1,2], see Fig. 1, or electron-positron created from vacuum [3]. Ultraintense laser pulses are applied to combine in one single-femtosecond stage the electron and positron acceleration and their microscopic coherent collision in the GeV regime. We have shown that such coherent collisions yield a largely enhanced luminosity compared to conventional incoherent colliders, so that particle physics reaction with high-power lasers become possible. As an example, the feasibility of muon pair production from a positronium gas in a strong laser field has been investigated, see Fig. 2. By investigating the laser-dressed polarization operator [3], we identify a new contribution describing high-energy recollisions experienced by an electron-positron pair generated by pure light when a gamma photon impinges on an intense, linearly polarized laser pulse. The energy absorbed in the recollision process over the macroscopic laser wavelength corresponds to a large number of laser photons and can be exploited to prime high-energy reactions.
[1] B. Henrich, K. Z. Hatsagortsyan and C. H. Keitel, Phys. Rev. Lett. 93, 013601 (2004).
[2] K. Z. Hatsagortsyan, C. Müller, and C. H. Keitel, Europhys. Lett. 76, 29 (2006).