Extreme Field Quantum Plasma Dynamics and Relativistic Laboratory Astrophysics
Relativistic quantum plasmas like gamma-electron-positron plasmas are believed to play a key role in extreme astrophysical environments such as pulsar magnetospheres, magnetars, black holes and blazars, and are central to explain energetic phenomena associated to gamma-ray bursts. Due to the complete symmetry between matter and antimatter particles, electron-positron plasmas represent a unique state qualitatively different from ordinary electron-ion plasmas. This implies that the collective response and the related instabilities of relativistic electron-positron plasmas result in a peculiar dynamics, whose knowledge is still speculative.
Recent advances in high-power laser and in high-current electron beam technology enable the generation in the laboratory of ultra-strong electromagnetic fields that can trigger strong-field QED processes such as photon emission and high-energy photon conversion into an electron-positron pair. This paves the way to generate and probe in the laboratory gamma-electron-positron plasmas similar to those of astrophysical environments, and justifies the flourishing interest in this area of research known as relativistic laboratory astrophysics.
Our research focuses on the investigation of the interplay between strong-field QED processes and the collective plasma dynamics in the presence of extreme electromagnetic fields. Prominent examples are radiation reaction effects in plasmas, where the recoil due to photon emissions drastically alters the emitting particle’s dynamics, the generation of intense collimated gamma rays and of dense high-energy spin-polarized particle beams in laser-plasma and beam-plasma interaction, QED cascades with the transformation of electromagnetic energy into matter, relativistic laboratory astrophysics. Because of the complex interplay between strong-field QED and the nonlinear plasma dynamics, large scale simulations constitute an essential tool. The development both of advanced models of strong-field QED processes and of improved numerical methods as well as their implementation into codes capable to accurately and efficiently simulate strong-field QED dominated plasmas in modern high-performance computing systems also represents one of our key activities.
People
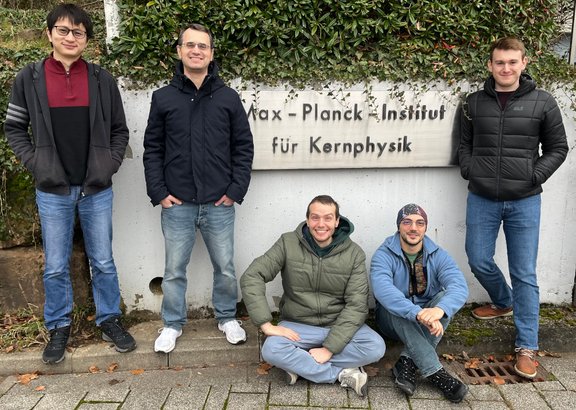
- Matteo Tamburini (Group Leader)
- Samuele Montefiori (Ph.D. student)
- Michael Quin (Ph.D. student)
- Yutong He (Ph.D. student)
- Cagri Erciyes (Ph.D. student)
Completed thesis and former team members
Apr. 2021 | Congratulations to Bastian Brunner for successfully defending his Bachelor thesis on "Electron Dynamics Controlled via Radiation Reaction". |
Feb. 2021 | Congratulations to Maitreyi Sangal for successfully defending her Ph.D. thesis on "The interaction of Light with Matter and Light with Light". |
Nov. 2020 | Congratulations to Archana Sampath for successfully defending her Ph.D. thesis on "Strong-field QED and collisional effects in electron beam-plasma interaction". |
Oct. 2020 | Congratulations to Michael Quin for successfully defending his Master thesis (fast track) on "Coherence Effects and Spin Polarisation of Electrons in Electromagnetic Fields". |
Observing light-by-light scattering in vacuum with an asymmetric photon collider
The elastic scattering of two real photons in vacuum is one of the most elusive of the fundamentally new processes predicted by QED. This explains why, although it was first predicted more than eighty years ago, it has so far remained undetected. We have shown that in present-day facilities, the elastic scattering of two real photons can become detectable far off axis in an asymmetric photon-photon collider setup [1]. This may be obtained within one day of operation time by colliding 1 mJ extreme ultraviolet pulses with the broadband gamma-ray radiation generated in nonlinear Compton scattering of ultrarelativistic electron beams with terawatt-class optical laser pulses operating at a 10 Hz repetition rate. In addition to the investigation of elastic photon-photon scattering, this technique allows us to unveil or constrain new physics that could arise from the coupling of photons to yet undetected particles which, for example, would result in a measurable signal differing from the QED prediction, therefore opening new avenues for searches of physics beyond the standard model of particle physics.
[1] M. Sangal, C. H. Keitel, and M. Tamburini, Phys. Rev. D 104, L111101 (2021)
Efficient high-energy photon production via beam-beam collision in the supercritical QED regime
The state-of-the-art concept to generate high-energy photons is based on Compton backscattering of an intense laser pulse with a counterpropagating electron beam. However, Compton backscattering becomes increasingly more challenging to realize with increasing center-of-mass energy because high-energy photons convert into electron-positron pairs inside the laser pulse. When dense high-energy lepton bunches collide, the beam particles can experience rest-frame electromagnetic fields which greatly exceed the QED critical one therefore emitting high-energy radiation via beamstrahlung. We have demonstrated that beamstrahlung efficiently converts lepton energy to high-energy photons in this so-called supercritical QED regime, as the single-photon emission spectrum exhibits a pronounced peak close to the initial lepton energy [1]. Remarkably, this high-energy peak is visible uniquely when (i) the rest frame fields exceed tens of times the QED critical one, (ii) when leptons experience nearly the same fields, and (iii) when multiple photon emissions by the same particle are suppressed. Otherwise, particles experiencing inhomogeneous electromagnetic fields or emitting several lower energy photons both broaden and soften the emitted photon spectrum. The collision of shaped lepton beams enables all the three above conditions simultaneously. On the one hand, this setup allows one to measure the high-energy peak in the photon spectrum, the height of the peak being in a one-to-one correspondence with the instantaneous rest frame electric field at emission, therefore opening up precision studies of strong-field QED in the ultra-relativistic regime. On the other hand, the proposed setup provides decisive advantages for the realization of an efficient multi-TeV laserless gamma-gamma collider based on electron-electron collisions because it allows one to simultaneously suppress the lower energy background and to increase the probability of almost complete lepton-to-photon energy transfer [1].
[1] M. Tamburini and S. Meuren, Phys. Rev. D 104, L091903 (2021)
How to make a gamma-ray pulse with the density of a solid
The generation of high-energy, dense and collimated photon beams is of great interest both to fundamental and applied research. These beams enable studies of matterless photon-photon interactions ranging from matter-antimatter creation from light, light-by-light scattering and searches of possible new physics in the interaction of photons with yet undiscovered particles, to relativistic laboratory astrophysics studies. We have demonstrated [1] that a pulsed, ultrarelativistic electron beam crossing a sequence of thin aluminium foils both self-focuses, therefore increasing its density, and efficiently yields a collimated gamma-ray pulse with more gamma photons per unit volume than electrons in a solid. After passage of 20 foils, more than 30% of the electron beam energy is converted to gamma rays. This occurs because the strong electric and magnetic fields accompanying the ultrarelativistic electron beam are “back-reflected” as the beam crosses the foil surface. Similar to an electromagnetic wave colliding with a mirror, the reflected magnetic field is nearly the same as the beam magnetic field, whereas the reflected electric field has the same amplitude as that of the electron beam but opposite direction. As a result, at the foil surface the total electric field acting on the beam is nearly zero, while the total magnetic field is nearly doubled. This strong azimuthal magnetic field focuses the electron beam radially, and consequently triggers collimated high-energy photon emission. Based on these findings, an experiment (E-332 experiment) for the investigation of electron beam self-focusing, solid-density gamma-ray burst generation, and laserless strong-field QED was devised, successfully approved with maximal rating, and is currently underway at FACET-II [2].
Spin-polarized kiloampere electron beams from laser-plasma acceleration
High-flux polarized particle beams are of critical importance for the investigation of spin-dependent processes such as in searches of physics beyond the standard model as well as for scrutinizing the structure of solids and surfaces in material science. We have demonstrated that kiloampere polarized electron beams can be produced via laser-wakefield acceleration from a gas target [1]. We have developed a simple theoretical model for determining the electron beam polarization, and we have supported our model predictions with self-consistent three-dimensional particle-in-cell simulations that incorporate the spin dynamics. By appropriately choosing the laser and gas parameters, we have shown that the depolarization of electrons induced by the laser-wakefield-acceleration process can be as low as 10%. Compared to currently available sources of polarized electron beams, the flux of spin-polarized electrons is increased by 4 orders of magnitude, thereby opening up unprecedented studies of spin-dependent processes.
[1] Meng Wen, Matteo Tamburini, and Christoph H. Keitel, Phys. Rev. Lett. 122, 214801 (2019)