Correlated and X-Ray Quantum Dynamics
Quantum optical concepts based on coherence and interference have proven extremely successful for the study and manipulation of atoms and molecules. Recent improvements in modern x-ray light sources prompt the question, whether such techniques could also be applied in the hard x-ray regime. This could not only pave the way for new applications, but also is indispensable for taking advantage of the full potential of these new machines.
However, despite the new light sources, it remains challenging to exploit quantum optical phenomena in the x-ray domain, and it is typically not possible to directly transfer established concepts to the x-ray domain. We develop new approaches to establish quantum optical methods at x-ray energies, focusing in particular on the coupling of x-rays to nuclear resonances in large ensembles of Mössbauer nuclei. While we mainly work theoretically, we regularly conduct experiments at x-ray sources in collaboration with other groups to explore new regimes and to verify our predictions.
Interestingly, the relevant setups in x-ray quantum optics typically involve strong collective phenomena inducing correlations within the ensemble of nuclei, and the understanding or even control of these phenomena is a key requirement. Motivated by this, we also study correlations and collective phenomena in other related model systems, as well as fundamental coherence and interference effects.
Certifying multi-mode light-matter interaction in lossy resonators
Quantum models based on few-mode Master equations have been a central tool in the study of resonator quantum electrodynamics, extending the seminal single-mode Jaynes-Cummings model to include loss and multiple modes. Despite their broad application range, previous approaches within this framework have either relied on a Markov approximation or a fitting procedure. By combining ideas from pseudomode and quasimode theory, we develop a certification criterion for multi-mode effects in lossy resonators without the need for a fitting procedure or a Markov approximation. Using the resulting criterion, we demonstrate that such multi-mode effects are important for understanding previous experiments in X-ray cavity QED with Mössbauer nuclei and that they allow one to tune the nuclear ensemble properties.
Unraveling Time-and Frequency-Resolved Nuclear Resonant Scattering Spectra
Owing to their extremely narrow line-widths and exceptional coherence properties, Mössbauer nuclei form a promising platform for quantum optics, spectroscopy and dynamics at energies of hard x-rays. A key requirement for further progress is the development of more powerful measurement and data analysis techniques. As one approach, recent experiments have employed time- and frequency-resolved measurements, as compared to the established approaches of measuring time-resolved or frequency-resolved spectra separately. In these experiments, the frequency-dependence is implemented using a tunable single-line nuclear reference absorber. Here, we develop spectroscopy and analysis techniques for such time- and frequency-resolved Nuclear Resonant Scattering spectra in the frequency-frequency domain. Our approach is based on a Fourier-transform of the experimentally accessible intensities along the time axis, which results in complex-valued frequency-frequency correlation (FFC) spectra. We show that these FFC spectra not only exhibit a particularly simple structure, disentangling the different scattering contributions, but also allow one to directly access nuclear target properties and the complex-valued nuclear resonant part of the target response. In a second part, we explore the potential of an additional phase control of the x-rays resonantly scattered off of the reference absorber for our scheme. Such control provides selective access to specific scattering pathways, allowing for their separate analysis without the need to constrain the parameter space to certain frequency or time limits. All results are illustrated with pertinent examples in Nuclear Forward Scattering and in reflection off of thin-film x-ray cavities containing thin layers of Mössbauer nuclei.
Parametric Mössbauer radiation source
Numerous applications of Mössbauer spectroscopy are related to a unique resolution of absorption spectra of resonant radiation in crystals, when the nucleus absorbs a photon without a recoil. However, the narrow nuclear linewidth renders efficient driving of the nuclei challenging, restricting precision spectroscopy, nuclear inelastic scattering and nuclear quantum optics. Moreover, the need for dedicated x-ray optics restricts access to only few isotopes, impeding precision spectroscopy of a wider class of systems. Here, we put forward a novel Mössbauer source, which offers resonant photon flux for a large variety of Mössbauer isotopes with strongly suppressed electronic background. It is based on relativistic electrons moving through a crystal and emitting parametric Mössbauer radiation essentially unattenuated by electronic absorption. As a result, a collimated beam of resonant photons is formed, without the need for additional monochromatization. We envision the extension of high-precision Mössbauer spectroscopy to a wide range of isotopes at accelerator facilities, also using dumped electron beams.
Inverse design in nuclear quantum optics
Ensembles of Mössbauer nuclei embedded in thin-film cavities form a promising platform for x-ray quantum optics. A key feature is that the joint nuclei-cavity system can be considered as an artificial x-ray multilevel scheme in the low-excitation regime. Using the cavity environment, the structure and parameters of such level schemes can be tailored beyond those offered by the bare nuclei. However, so far, the direct determination of a cavity structure providing a desired quantum optical functionality has remained an open challenge. Here we address this challenge using an inverse design methodology. We show that the established fitting approach based on scattering observables in general is not unique, since the analysis may lead to different multilevel systems for the same cavity if based on observables in different scattering channels. Motivated by this, we distinguish between scattering signatures and the microscopic level scheme as separate design objectives, with the latter being uniquely determined by an ab initio approach. We find that both design objectives are of practical relevance and that they complement each other regarding potential applications. We demonstrate the inverse design for both objectives using example tasks, such as realizing electromagnetically induced transparency. Our results pave the way for future applications in nuclear quantum optics involving more complex x-ray cavity designs.
Coherent X-ray-optical control of nuclear excitons
Coherent control of quantum dynamics is key to a multitude of fundamental studies and applications. In the visible or longer-wavelength domains, near-resonant light fields have become the primary tool with which to control electron dynamics. Recently, coherent control in the extreme-ultraviolet range was demonstrated, with a few-attosecond temporal resolution of the phase control. At hard-X-ray energies (above 5–10 kiloelectronvolts), Mössbauer nuclei feature narrow nuclear resonances due to their recoilless absorption and emission of light, and spectroscopy of these resonances is widely used to study the magnetic, structural and dynamical properties of matter. It has been shown that the power and scope of Mössbauer spectroscopy can be greatly improved using various control techniques. However, coherent control of atomic nuclei using suitably shaped near-resonant X-ray fields remains an open challenge. Here we demonstrate such control, and use the tunable phase between two X-ray pulses to switch the nuclear exciton dynamics between coherent enhanced excitation and coherent enhanced emission. We present a method of shaping single pulses delivered by state-of-the-art X-ray facilities into tunable double pulses, and demonstrate a temporal stability of the phase control on the few-zeptosecond timescale. Our results unlock coherent optical control for nuclei, and pave the way for nuclear Ramsey spectroscopy and spin-echo-like techniques, which should not only advance nuclear quantum optics, but also help to realize X-ray clocks and frequency standards. In the long term, we envision time-resolved studies of nuclear out-of-equilibrium dynamics, which is a long-standing challenge in Mössbauer science.
- K. P. Heeg, A. Kaldun, C. Strohm, C. Ott, R. Subramanian, D. Lentrodt, J. Haber, H. Wille, S. Goerttler, R. Rüffer, C. H. Keitel, R. Röhlsberger, T. Pfeifer, and J. Evers, Nature 590, 401-404 (2021)
- MPIK press release: X-ray double flashes control atomic nuclei
- MPG press release: Atomic nuclei in the quantum swing
- Selection to the Physics World Top 10 Breakthroughs 2021
Ab initio quantum models for thin-film x-ray cavity QED
We develop two ab initio quantum approaches to thin-film x-ray cavity quantum electrodynamics with spectrally narrow x-ray resonances, such as those provided by Mössbauer nuclei. The first method is based on a few-mode description of the cavity, and promotes and extends existing phenomenological few-mode models to an ab initio theory. The second approach uses analytically known Green's functions to model the system. The two approaches not only enable one to ab initio derive the effective few-level scheme representing the cavity and the nuclei in the low-excitation regime, but also provide a direct avenue for studies at higher excitation, involving nonlinear or quantum phenomena. The ab initio character of our approaches further enables direct optimizations of the cavity structure and thus of the photonic environment of the nuclei, to tailor the effective quantum optical level scheme towards particular applications. To illustrate the power of the ab initio approaches, we extend the established quantum optical modeling to resonant cavity layers of arbitrary thickness, which is essential to achieve quantitative agreement for cavities used in recent experiments. Further, we consider multilayer cavities featuring electromagnetically induced transparency, derive their quantum optical few-level systems ab initio, and identify the origin of discrepancies in the modeling found previously using phenomenological approaches as arising from cavity field gradients across the resonant layers.
Phase-sensitive nuclear target spectroscopy
Mössbauer nuclei feature exceptionally narrow resonances at hard x-ray energies, which render them ideal probes for structure and dynamics in condensed-matter systems, and a promising platform for x-ray quantum optics and fundamental tests. However, a direct spectroscopy at modern x-ray sources such as synchrotrons or x-ray free-electron lasers is challenging, because of the broad spectral bandwidth of the delivered x-ray pulses, and because of a limited spectral resolution offered by x-ray optics and detectors. To overcome these challenges, here, we propose a spectroscopy technique based on a spectrally narrow reference absorber that is rapidly oscillating along the propagation direction of the x-ray light. The motion induces sidebands to the response of the absorber, which we scan across the spectrum of the unknown target to gain spectral information. The oscillation further introduces a dependence of the detected light on the motional phase at the time of x-ray excitation as an additional controllable degree of freedom. We show how a Fourier analysis with respect to this phase enables one to selectively extract parts of the recorded intensity after the actual experiment, throughout the data analysis. This allows one to improve the spectral recovery by removing unwanted signal contributions. Our method is capable of gaining spectral information from the entire measured intensity, and not only from the intensity at late times after the excitation, such that a significantly higher part of the signal photons can be used. Furthermore, it not only enables one to measure the amplitude of the spectral response but also its phase.
Ab Initio Few-Mode Theory for Quantum Potential Scattering Problems
Few-mode models are a cornerstone of the theoretical work in quantum optics, with the famous single-mode Jaynes-Cummings model being only the most prominent example. In this work, we develop an ab initio few-mode theory, a framework connecting few-mode system-bath models to ab initio methods. We first present a method to derive exact few-mode Hamiltonians for noninteracting quantum potential scattering problems and demonstrate how to rigorously reconstruct the scattering matrix from such few-mode Hamiltonians. We show that, upon inclusion of a background scattering contribution, an ab initio version of the well-known input-output formalism is equivalent to standard scattering theory. On the basis of these exact results for noninteracting systems, we construct an effective few-mode expansion scheme for interacting theories, which allows us to extract the relevant degrees of freedom from a continuum in an open quantum system. As a whole, our results demonstrate that few-mode as well as input-output models can be extended to a general class of problems and open up the associated toolbox to be applied to various platforms and extreme regimes. We outline differences of the ab initio results to standard model assumptions, which may lead to qualitatively different effects in certain regimes. The formalism is exemplified in various simple physical scenarios. In the process, we provide a proof of concept of the method, demonstrate important properties of the expansion scheme, and exemplify new features in extreme regimes.
Time-Resolved sub-Ångström Metrology by Temporal Phase Interferometry near X-Ray Resonances of Nuclei
We introduce an analytical phase-reconstruction principle that retrieves atomic scale motion via time-domain interferometry. The approach is based on a resonant interaction with high-frequency light and does not require temporal resolution on the time scale of the resonance period. It is thus applicable to hard x rays and γ rays for measurements of extremely small spatial displacements or relative-frequency changes. Here, it is applied to retrieve the temporal phase of a 14.4 keV emission line of an 57Fe sample, which corresponds to a spatial translation of this sample. The small wavelength of this transition (λ=0.86 Å) allows for determining the motion of the emitter on sub-Ångström length and nanosecond timescales.
Probing quantum dynamical couple correlations with time-domain Interferometry
Time-domain interferometry (TDI) is a promising method to characterize spatial and temporal correlations at x-ray energies, via the so-called intermediate scattering function and the related dynamical couple correlations. However, so far, it has only been analyzed for classical target systems. Here, we provide a quantum analysis, and suggest a scheme that allows us to access quantum dynamical correlations. We further show how TDI can be used to exclude classical models for the target dynamics, and illustrate our results using a single particle in a double well potential. Interestingly, QTDI can access the full quantum-mechanical two-time correlations without backaction.
Spectral narrowing of x-ray pulses for precision spectroscopy with nuclear resonances
Spectroscopy of nuclear resonances offers a wide range of applications due to the remarkable energy resolution afforded by their narrow linewidths. However, progress toward higher resolution is inhibited at modern x-ray sources because they deliver only a tiny fraction of the photons on resonance, with the remainder contributing to an off-resonant background. We devised an experimental setup that uses the fast mechanical motion of a resonant target to manipulate the spectrum of a given x-ray pulse and to redistribute off-resonant spectral intensity onto the resonance. As a consequence, the resonant pulse brilliance is increased while the off-resonant background is reduced. Because our method is compatible with existing and upcoming pulsed x-ray sources, we anticipate that this approach will find applications that require ultranarrow x-ray resonances.
Full inversion of nuclear ensembles with x-ray free electron lasers
Up to now, experiments involving Mössbauer nuclei driven by x-rays have been restricted to the low-excitation regime. To overcome this problem, we recently proposed a setup which promises significant excitation, ideally exceeding full inversion of the nuclear ensemble, at x-ray light sources under construction. We further introduced a method to experimentally verify such inversions, in which population inversions manifest themselves in symmetry flips of suitably recorded spectra. It neither requires per-shot spectra of the incoming x-ray pulses, nor absolute measurements of the scattered light intensity. We also estimated the effect of the pulse-to-pulse coherence in x-ray free electron laser oscillators, and showed that it could lead to orders of magnitude enhancement of the signal rate for ultra-narrow nuclear resonances. These are of primary significance for precision spectroscopy, metrology, and fundamental tests.
Tailoring superradiance to design artificial quantum systems
Cooperative phenomena arising due to the coupling of individual atoms via the radiation field are a cornerstone of modern quantum and optical physics. Recent experiments on x-ray quantum optics added a new twist to this line of research by exploiting superradiance in order to construct artificial quantum systems. However, so far, systematic approaches to deliberately design superradiance properties are lacking, impeding the desired implementation of more advanced quantum optical schemes. To overcome this issue, we developed an analytical framework for the engineering of single-photon superradiance in extended media. Our approach is applicable to a large class of model systems across the entire electromagnetic spectrum. The key idea is to use an ensemble of atoms with fixed properties. By controlling the cooperative dynamics of the ensemble, a tunable artificial quantum system is created. Our analytic approach establishes a one-to-one relation between artificial atom and ensemble. This way, superradiance can be reverse engineered, which provides an avenue towards non-linear and quantum mechanical phenomena at x-ray energies, and also leads to a unified view on and a better understanding of superradiance across different physical systems.
A new phase for x-ray quantum optics
Modern x-ray light sources promise access to structure and dynamics of matter in largely unexplored spectral regions. However, the desired information is encoded in the light intensity and phase, whereas detectors register only the intensity. This phase problem is ubiquitous in crystallography and imaging and impedes the exploration of quantum effects at x-ray energies. In this experiment, we demonstrated phase-sensitive measurements characterizing the quantum state of a nuclear two-level system at hard x-ray energies. The nuclei are initially prepared in a superposition state. Subsequently, the relative phase of this superposition is interferometrically reconstructed from the emitted x rays. Our results form a first step towards x-ray quantum state tomography and provide new avenues for structure determination and precision metrology via x-ray Fano interference.
A handbrake for x-rays
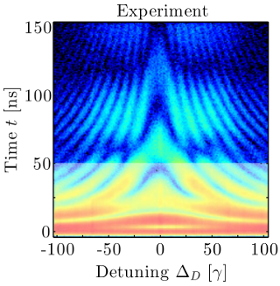
We experimentally demonstrated group velocity control for x-ray photons of 14.4 keV energy via a direct measurement of the temporal delay imposed on spectrally narrow x-ray pulses. The subluminal light propagation was achieved by inducing a steep positive linear dispersion in the optical response of Fe Mössbauer nuclei embedded in a thin film planar x-ray cavity. To directly detect the temporal pulse delay, we developed a new method to generate frequency-tunable spectrally narrow x-ray pulses from broadband pulsed synchrotron radiation. Our theoretical model is in good agreement with the experimental data. This project is an important step towards the exploitation of non-linear phenomena with nuclei.
Quantum states out of nothing
Quantum mechanical superpositions are important resources for future quantum technologies, but extremely fragile: already the interaction with the vacuum alone can destroy them. A promising solution in theory is already known for more than 40 years. At that time it was predicted that the interaction with the vacuum may be manipulated in such a way that it produces the desired superpositions instead of destroying them. Unfortunately, this is linked to rigorous conditions which so far hindered the experimental realization. We have circumvented these constraints, and demonstrated the spontaneous generation of coherences between the quantum states of a large ensemble of nuclei. Probing the system using nuclear resonance scattering, we observed clear signatures of the formed coherences, controlled by a weak external magnetic field. This opens up a variety of future perspectives for quantum optics with novel X-ray light sources.
Theory of x-ray cavity quantum electrodynamics
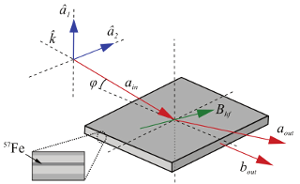
Mössbauer nuclei embedded in thin-film cavities have proven to be a most promising platform for x-ray quantum optics, as evidenced by a number of recent experiments. We developed a comprehensive quantum optical framework for the description of experimentally relevant settings involving nuclei embedded in x-ray waveguides. For a large class of experimentally relevant conditions, we derived compact analytical expressions, and showed that the alignment of medium magnetization, as well as incident and detection polarization, enable the engineering of advanced quantum optical level schemes. The model encompasses nonlinear and quantum effects, and we have recently also extended it to encompass multiple nuclear ensembles and multiple cavity modes as used in some experiments. Our theoretical model has been successfully employed to design and quantitatively analyse several recent experiments.
Self-assembly of Rydberg aggregates
In strongly interacting Rydberg gases, the atom-atom interactions induce level shifts for multi-particle excitation states. At off-resonant laser driving, the laser detuning can compensate these level shifts, leading to resonant excitation channels for multi-particle Rydberg aggregates. We study such off-resonant driving in a cloud of ultracold two-level atoms. We find that resonant excitation channels lead to strongly peaked spatial correlations associated with the buildup of asymmetric excitation structures. These aggregates can extend over the entire ensemble volume, but are in general not localized relative to the system boundaries. The characteristic distances between neighboring excitations within an aggregate depend on the laser detuning and on the interaction potential. These properties lead to unique features in the spatial excitation density, the Mandel Q parameter, and the total number of excitations. We have also modeled recent related experiments in a strongly dissipative Rydberg system.
- M. Gärttner, K. P. Heeg, T. Gasenzer, and J. Evers, Phys. Rev. A 88, 043410 (2013)
- H. Schempp, G. Günter, M. Robert-de-Saint-Vincent, C. S. Hofmann, D. Breyel, A. Komnik, D. W. Schönleber, M. Gärttner, J. Evers, S. Whitlock, M. Weidemüller, Phys. Rev. Lett. 112, 013002 (2014)
- K. Heeg, M. Gärttner, and J. Evers, Phys. Rev. A 86, 063421 (2012)
Rydberg electromagnetically induced transparency
Highly excited Rydberg atoms have extreme properties, which make them promising candidates for a number of fascinating applications. Most importantly, they feature long-range interactions, which lead to correlated dynamics of the ensemble of atoms. One application is to achieve strong interactions between photons by interfacing them with interacting states of matter. Recently, electromagnetically induced transparency was implemented in a cold gas of Rydberg atoms. The strong interaction of the Rydberg atoms leads to nonlinear optical effects such as a nontrivial dependence of the degree of probe-beam attenuation on the medium density and on its initial intensity. Furthermore, so-called dark-state polaritons form, which are combined excitation of the photon field and the atoms. Due to the atom-atom interactions, the polariton number statistics is modified to non-classical values. We have developed a Monte Carlo rate equation model to describe this setting, which self-consistently includes the effect of the probe-beam attenuation to investigate the steady state of the Rydberg medium driven by two laser fields. This model successfully describes the optical response observed in a recent experiment.
Single-photon entanglement in the hard x-ray regime
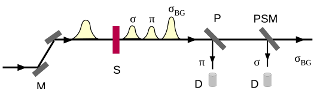
Quantum entanglement is one of the most intriguing properties of quantum mechanics, and is commonly visualized as a correlation of two or more quantum particles that form a single quantum object. But entanglement does not necessarily involve two quantum particles. Possibly even more fascinating, a single photon can entangle two modes of the vacuum. Here we show that this single-particle entanglement can also be generated by coherently controlling the quantum dynamics of nuclei. First, the nuclei are excited with a synchrotron radiation pulse. Then, by applying a sequence of weak magnetic fields to the nuclei, they are forced to emit a single photon in the x-ray regime such that it entangles field modes at energies about 10000 times higher than usual. This could pave the way for entanglement research in nuclear physics, based on experiments exploiting nuclear complexity with synchrotron radiation sources and free-electron lasers in an as-yet unexplored energy regime.
- A. Pálffy, C. H. Keitel, and J. Evers, Phys. Rev. Lett. 103, 017401 (2009)
(and July 2009 issue of Virtual Journal of Quantum Information)
(and July 13, 2009 issue of Virtual Journal of Nanoscale Science & Technology)
Isomer triggering via nuclear excitation by electron capture
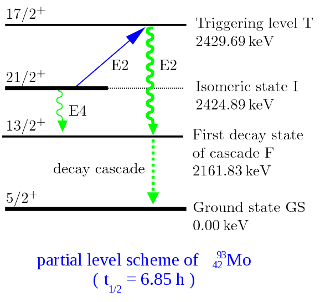
The search for practical methods to change the internal state of atomic nuclei has been the subject of a number of investigation in the last years. In particular, isomer triggering refers to the possibility to excite the long-lived excited isomeric nuclear state to a higher level which is associated with freely radiating states and therefore releases the energy of the metastable state. Isomers are of interest in different contexts, for example, due to fascinating potential applications related to the controlled release of nuclear energy on demand, such as in nuclear batteries, or motivated by the fundamental challenge to understand the formation of isomers and their role in the evolution of the universe.
We have compared isomer triggering via photoabsorption to low-lying triggering levels with alternative mechanisms. We show that x-ray triggering is possible, but it turns out that among the possible isomer triggering mechanisms, the coupling to the atomic shell via the process of nuclear excitation by electron capture is the most efficient one. An experimental verification of our findings at the borderline of atomic and nuclear physics may be provided by upcoming ion storage ring facilities and ion beam traps which will commence operation in the near future.
Nuclear quantum optics with x-ray laser pulses
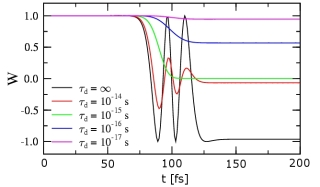
The direct interaction of nuclei with super-intense laser fields is studied. We show that present and upcoming high-frequency laser facilities, especially together with a moderate acceleration of the target nuclei to match photon and transition frequency, do allow for resonant laser-nucleus interaction. These direct interactions may be utilized for the model-independent optical measurement of nuclear properties such as the transition frequency and the dipole moment, thus opening the field of nuclear quantum optics. As ultimate goal, one may hope that direct laser-nucleus interactions could become a versatile tool to enhance preparation, control and detection in nuclear physics.