Exotic Quantum Systems
One of the main research directions of the group is the theoretical study of exotic atomic systems, in particularly muonic atoms. They feature strong dependence on nuclear parameters and therefore can provide information about atomic nuclei. Since muonic atoms can be treated as hydrogen-like highly charged ions, a well-known theoretical QED methods are applicable here, however with the much higher impact of the nuclear physics. A combination of the knowledge about the level structure and experiments measuring the transition energies in muonic atoms enabled the determination of nuclear parameters like charge radii, electric quadrupole and magnetic dipole moments.
Another direction of our work is high-precision theoretical predictions, including nuclear structure effects based on microscopic theory. Such calculations are getting important for measurements aiming to the tests of existing theories, determination of fundamental constants, search for the new physics etc.
Finally, we performs many-body calculations for atoms and ions, to support nuclear-parameters determinations, simulations of dynamical effects in astrophysically relevant experiments, and other fundamental studies.
The group collaborates with few experimental groups around the world.
Group members
- PD Dr. Natalia S. Oreshkina (Group Leader)
- Dr. Igor A. Valuev (PostDoc)
- Dr. Konstantin Beyer (PostDoc)
- Zewen Sun (PhD student)
- Zoia Mandrykina (PhD student)
Moritz Thierfeld (BSc student)
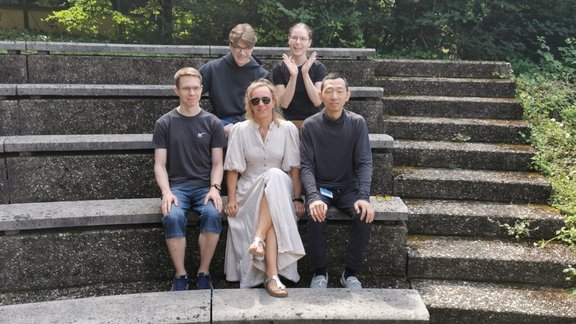

Recent projects
1) High-precision theory for heavy muonic atoms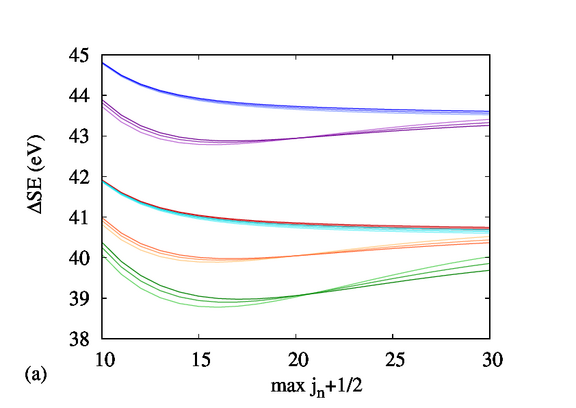
When coming close to an atom, a muon can be captured by the nucleus and form a hydrogen-like muonic ion, which is typically also surrounded by atomic electrons. This atomic system is commonly referred to as a muonic atom. The lifetime of the muon is large enough to be considered stable in the structure calculations of these muonic bound states. Muonic atomic systems feature strong dependence on nuclear parameters and therefore can provide information about atomic nuclei. This triggered interest in precise knowledge of the level structure of muonic atoms. Due to the muon’s high mass, it is located much closer to the nucleus; and, especially for heavy nuclei, this results in big nuclear size effects and a strong dependence of the muon bound-state energies on the nuclear charge and current distributions, as well as large relativistic effects. A combination of the knowledge about the level structure and experiments measuring the transition energies in muonic atoms enabled the determination of nuclear parameters like charge radii, electric quadrupole and magnetic dipole moments.
With our paper Ref. [1] we have started the improved state-of-the-art calculations of the fine and hyperfine structures, and QED effects of heavy muonic atoms. The most significant finite-size corrections, leading-order vacuum polarization, electron screening, and nonrelativistic recoil corrections were included already in the solution of the Dirac equation. Thus, all further calculations also contain these effects by using the corrected wave functions. In Ref. [2] the dynamical splitting, including higher-order corrections, in heavy muonic atoms has been calculated. We presented a method for precise calculation of the energy corrections due to second-order electric-as well as mixed electric quadrupole-vacuum polarization. These calculations have been performed using a deformed Fermi distribution. In Ref. [3] we have combined our theory predictions with a measured experimental spectra of two rhenium isotopes to extract new values of nuclear quadrupole moment. Finally, the recent work [4] represent the last missing QED contribution to the muonic energy levels, namely, first results for rigorous self-energy calculations.
[1] N. Michel, N. S. Oreshkina, and C. H. Keitel, Phys. Rev. A 96, 032510 (2017)
[2] N. Michel and N. S. Oreshkina, Phys. Rev. A 99, 042501 (2019)
[3] A. Antognini, N. Berger, T. E. Cocolios et al., Phys. Rev. C 101, 054313 (2020)
[4] N. S. Oreshkina, Phys. Rev. Research 4, L042040 (2022)
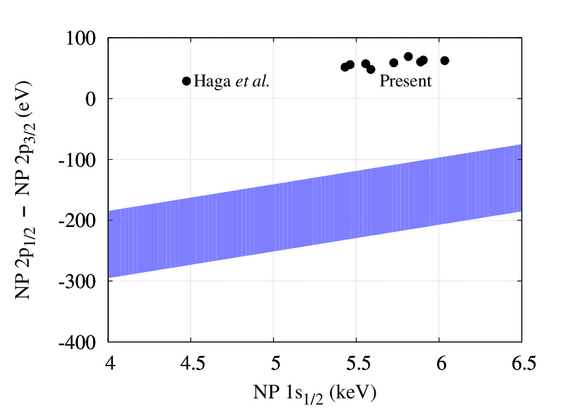
To improve even further our knowledge on the nuclear effects and to improve their accuracy in high-precision calculations, we use a detailed description of nuclear dynamics. First, the nuclear ground-state charge distributions obtained from the Skyrme-Hartree-Fock procedure has used to calculate the finite-nuclear-size correction to atomic energy levels and the bound-electron g factor in Ref. [5]. Then, a detailed description of nuclear dynamics is incorporated into calculations of the nuclear-polarization corrections to energy levels of muonic atoms in Ref. [6]. Nuclear ground-state charge distributions are obtained within the Hartree-Fock method, while complete nuclear excitation spectra are computed by means of the random-phase approximation. The effects of nuclear excitations on atomic properties are described in a field-theoretical framework. Special attention is given to analyzing the nuclear model dependence, and the uncertainties of the calculations are estimated. Finally, the developed methods and computational codes were applied to the long-standing problem of the fine-structure anomalies in heavy muonic atoms. Our results show that nuclear polarization effect is unlikely to be responsible for the anomaly, despite over 40 years believes.
In the work Ref. [7] we went even further with the complexity, and have calculated the nuclear-polarization effects to both g-factor and hyperfine structure of hydrogen-like 3He ion, the atomic system which have been proposed as a new standard for absolute magnetometry. This was an important contribution to the improved theoretical prediction, and in the combination with over order-of-magnitude improvement measurements, it allowed to determine Zemach radius to below 1% accuracy.
[5] I. Valuev, Z. Harman, C. H. Keitel, and N. S. Oreshkina, Phys. Rev. A 101, 062502 (2020)
[6] I. A. Valuev, G. Colò, X. Roca-Maza, C. H. Keitel, and N. S. Oreshkina, Phys. Rev. Lett. 128, 203001 (2022)
[7] A. Schneider, B. Sikora, S. Dickopf et al., Nature 606, 878-883 (2022)
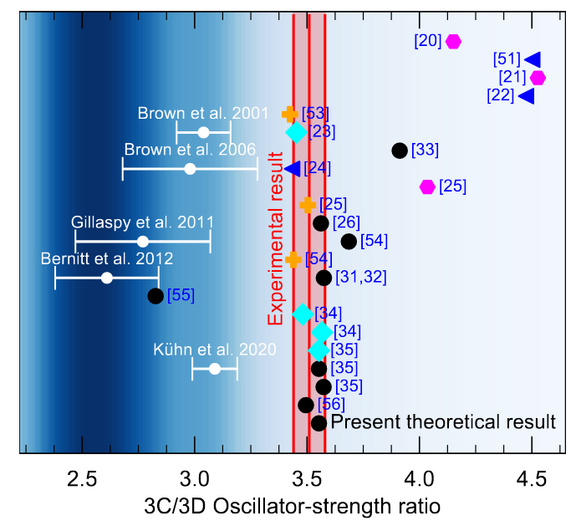
Astrophysical spectra recorded by space observatories provide the only means to determine the element composition, temperature, density, and velocity of distant celestial objects such as stars, x-ray binaries, black-hole accretion disks, or active galactic nuclei. Such spectra are often composed of a series of peaks associated with a range of elements, ionic charge states, and transitions. Therefore, a large amount of reliable atomic data is needed to disentangle the physical properties of the emitting objects. The x-ray emission lines of highly charged Fe ions, in particular Fe16+, are among the brightest in astrophysical spectra.
The calculated x-ray spectrum of Fe16+ is, however, not properly reproduced by astrophysical models. In particular, the line-strength ratio of two 2p → 3d lines, customarily denoted as 3C and 3D (at a transition energy of 826 and 812 eV, correspondingly) was observed, but astrophysical results for the 3C/3D line-strength ratio was lower than predicted values. Laboratory measurements employing a purely photonic excitation of the ions, gave hints for a shortcoming of atomic structure theory: a disagreement between all theoretical predictions (ratio of around 3.5 and above) and the experimental line-strength ratio of 2.61(23) has been stated. In our Ref. [8], motivated by the above discrepancy, we refine the theory of x-ray-ion interactions by calculating higher-order electron-correlation and dynamical effects contributing to the 3C/3D line-strength ratio. It suggests that the observed unexpectedly low laboratory value of the 3C/3D ratio is determined by previously neglected nonlinear dynamical effects, thus, it depends on the precise XFEL parameters. Therefore, to check this theory, a new experiment in a low-energy regime has been performed [9]. The result of this measurement allowed to exclude all proposed explanations, including those invoking nonlinear effects and population transfers. However, the disagreement of five sigmas between theory and experiment remained. Finally, the most recent work Ref. [10] at PETRA III synchrotron facility featured the two-and-a-half increasing of the resolving power and thousand-times improvement in signal-to noise-ratio, compared to the previous work [9]. The resulting experimental ratio finally agrees with all state-of-the-art theoretical predictions. This finally resolves one of the most enduring and intensively studied problems of X-ray astronomy.