GW 170817: Follow-up observations of a neutron-star neutron-star merger
November 2017
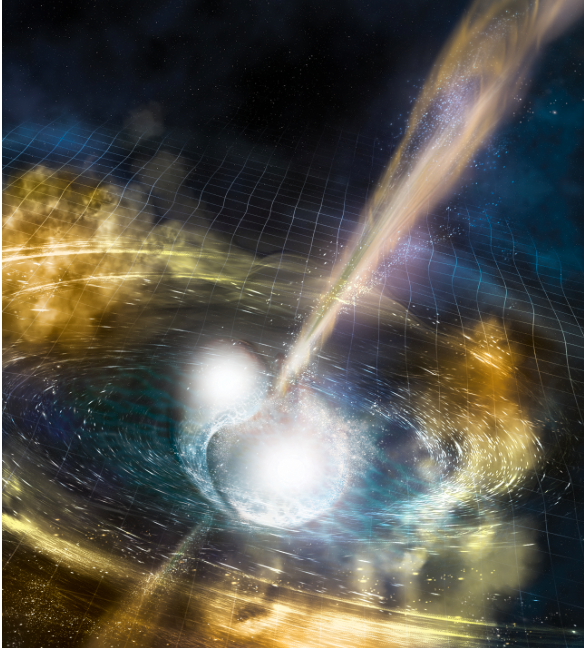
More than one hundred years have passed since Albert Einstein published his theory of general relativity, which has since proven to be one of the most groundbreaking scientific milestones of the 20th century. Through the warping of space-time this very successful theory introduced, one is able to correctly describe effects like gravitational lensing, orbital procession of elliptical orbits, and gravitational wave emission.
About 43 years have passed since Hulse and Taylor, when studying the relativistic orbit corrections of a binary system known as PSR 1913+16, discovered that the two components were approaching each other with time [1]. The system consists of a pair of neutron stars, one of them being a pulsar, that are inspiralling at an increasing rate, an effect that could be explained by energy loss due to gravitational wave emission. This pioneering work was awarded with the Nobel Prize in Physics in 1993 for the discovery of new possibilities to study gravitation. Note that this binary system will eventually merge, "eventually" here meaning within the next 300 million years!
Not willing to wait for PSR 1913+16 to observe a binary coalescence, the LIGO-Virgo collaboration, after long years of preparatory work, started started scanning the local universe for gravitational waves in 2015. Shortly after, the first black hole merger ever detected was observed by the LIGO interferometers [2]. The first detection of the coalescence of two neutron stars was observed two years later on August 17, 2017, only days after the Virgo interferometer joined the effort. This event is marking the beginning of gravitational wave multi-messenger astronomy (Fig. 1) [3].
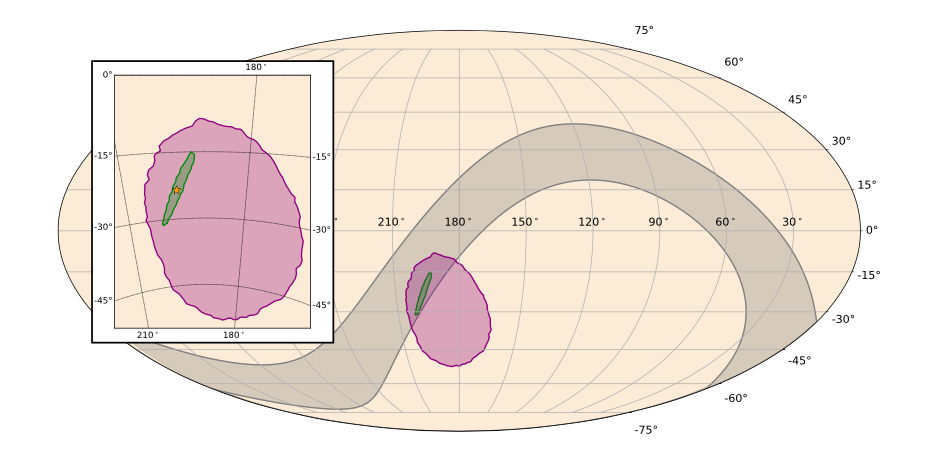
In general multi-wavelength and multi-messenger observations of the same astrophysical event provide us an enormous amount of valuable information, which cannot be provided by any one type of observation alone. While the gravitational waves provide us the masses, distance and the inclination of the initial binary system, electromagnetic detections give us information about the luminosity, redshift, duration and detailed properties of the system after the merger. Furthermore, the availability of many facilities studying the electromagnetic spectrum, from very high energies to the radio domain, enable extensive monitoring campaigns allowing to derive a complete description covering the different epochs of the system. This interplay of observatories around the world could for the first time be realized during the follow-up of the binary neutron star merger GW170817.
The gravitational wave event GW170817 (detected on August 17, 2017, and identified as a the first neutron star merger), was followed two seconds later by a short GRB, detected by the Gamma-ray Burst Monitor (GBM) mounted on the Fermi satellite. These two events triggered by far the most extensive follow-up campaign the worldwide astronomy and multi-messenger community has ever seen. A timeline of a subset of these observations are illustrated in Fig. 2.
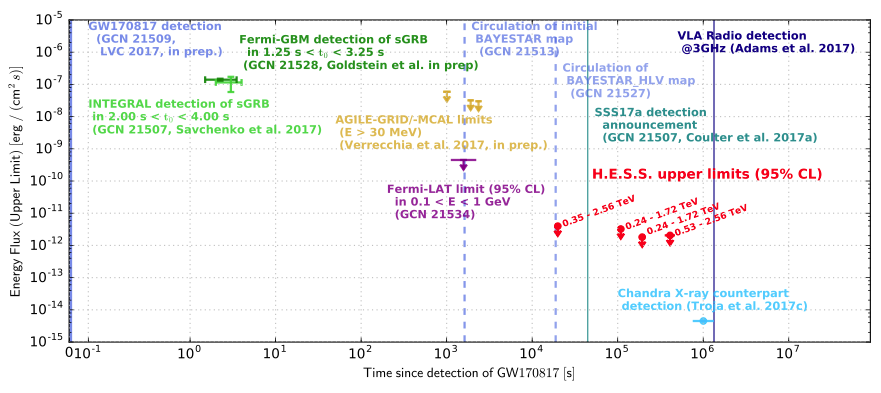
H.E.S.S. successfully participated in this campaign: observations started right after the night fall in the Namibian desert, only about 5 hours after the GW+GRB event. Observations focused on observing the most interesting sky locations, found by the convolution of the reconstruction of the gravitational wave event with a catalogue of galaxies [7]. Three observation runs were taken that night. Thanks to the focus on regions with a high density of galaxies compatible with the localisation of the GW event, the first observation covered the region in which the optical counterpart (aka kilonova) to the neutron star was detected several hours later. H.E.S.S. was thus the first ground-based instrument which obtained observations on this source. We did not detect any gamma-ray emission above an energy threshold of about 280GeV. Further observations were obtained during the following nights until the region was not visible anymore. The obtained upper limits on the gamma-ray flux (Fig. 3) constrain the non-thermal emission from this remarkable event.
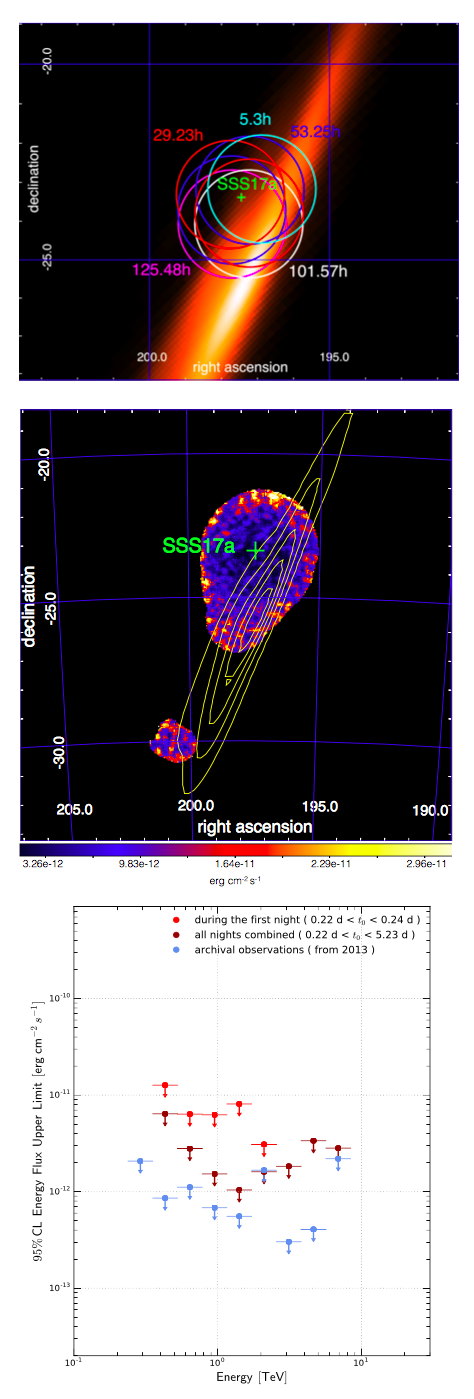
In summary, the multi-messenger campaign [5] led to the identification of the event as a binary neutron star merger, initiating a short gamma-ray burst and a blue-kilonova. The event was located in the galaxy NGC4993, at distance of ~ 40 Mpc (around 130 million light years). The distance of the event is much closer to us compared to any other GRB observed. It is also several orders of magnitudes less luminous. New experiments lead to new discoveries and challenges of our knowledge! In this case… the GRB event would certainly not have been followed up if no gravitational wave were involved. And it is only the extensive follow-up campaign that allowed to discover the associated kilonova. How many of these interesting events did we miss in the past? Or is this a first step away from the generic gamma-ray burst models and towards their description as individual astrophysical phenomena?
Even though these recent developments are very exciting on their own, they are likely just an appetizer of the many things to come in this new era of multi-messenger astronomy. Presently, improvements to the sensitivity are being carried out on the observatories that performed the gravitational wave observations, allowing them to get closer to their design sensitivity. Observations of the further improved LIGO and Virgo interferometers are scheduled for Fall 2018.
In the meantime, H.E.S.S. continues to improve its follow-up of transient events. Not only hardware-wise, with the upgrade of H.E.S.S. to H.E.S.S. II with a 28-m telescope in the center of the array, which allows to lower the energy threshold for extragalactic studies and reduce the slewing time, but also software-wise with the development of a general H.E.S.S. transient follow-up system, that includes an alert system based on the VOEvent standard [7] with a dedicated GW follow-up algorithm [6], a central control system being able to react fully automatically on alerts, and a real-time analysis. A suite of vital tools for multi-messenger studies that make sure we don't miss any of the interesting events and are able to play a central role in the new field of time-domain astronomy. Exciting discoveries ahead!
Stay tuned!
References:
H. Abdalla et al. (H.E.S.S. Collaboration): "TeV gamma-ray observations of the binary
neutrion star merger GW170817 with H.E.S.S.", arXiv 1710.1710.05862,
submitted.
[1] J.H. Taylor, L.A. Fowler, J.M. Weisberg, Nature
277 (1979) 437
[2] LIGO Scientific Collaboration and Virgo
Collaboration, PRL 116 (2016) 061102
[3] LIGO Scientific Collaboration and Virgo
Collaboration, PRL 119 (2017) 161101
[4] B.D. Metzger, G. Martinez-Pinedo, S. Darbha, et
al., MNRAS 406 (2010) 2650
[5] LIGO Scientific Collaboration and Virgo
Collaboration, et al., The Astrophysical Journal Letters, 848 (2017)
12
[6] M. Seglar-Arroyo and F. Schüssler (for the
H.E.S.S. Collaboration),
Proc. Rencontres de Moriond VHEPU (2017), arXiv:1705.10138
[7] C. Hoischen et
al. (for the H.E.S.S. Collaboration), Proc. 35th ICRC (Busan, Korea),
arXiv:1708:01088