A large armada targets famous M87
May 2021
In April 2019 the Event Horizon Telescope (EHT) team presented the first image of the shadow of the supermassive black hole inside the famous galaxy M87 [1]. This spectacular result was made possible by joint observations of many radio telescopes around the globe. Their target, M87, is the brightest galaxy in the nearby Virgo Cluster. It was suspected to host the largest black hole in the sky - the best candidate for the first attempt to obtain an actual image of such an elusive object. The attempt was successful. The picture showed an asymmetric ring of light. Its size and shape are consistent with the expectations for a black hole of about 6.5 billion solar masses that accretes matter from its vicinity at a low rate.
Many supermassive black holes also emit gamma-rays of very high energies. M87 was actually one of the first objects discovered to emit such very-high-energy gamma rays after H.E.S.S. started its operations in 2004 H.E.S.S. SOM 11/2006. ``Where and how are gamma-rays produced?'' are key questions for high-energy astrophysics and they have been particularly difficult to answer in the case of M87. Given its proximity (in astronomical terms), this galaxy can be studied in close detail. The angular resolution in the optical and X-ray regimes – and in particular in the radio range that provides us with the superb EHT resolving power used to map out the black hole horizon – is much higher than achievable in the gamma-ray range. Gamma-ray observations however provided complementary insights. Along with the first detection of gamma rays from this source in 2005, the H.E.S.S. observations also revealed rapid variations, demonstrating that the gamma rays are emitted from a region within M87 that is as compact as the region now imaged as the shadow of the supermassive black hole.
This similarity supports the assumption that the origin of gamma ray emission is related to the enigmatic black hole. One way to confirm this speculation is to compare physical models with observations obtained in all energy bands. Given the variability, such measurements have to be obtained simultaneously.
The H.E.S.S. collaboration hence joined forces with many colleagues to complement the EHT campaign with contemporaneous observations. In parallel to the EHT observations, an extensive multi-wavelength campaign with ground- and space-based telescopes across the entire electromagnetic spectrum was carried out [2]. The images obtained by the telescopes at different wavelengths and angular resolutions are shown in Figure 1 and visualized in a corresponding video. The data were collected by a team of 760 scientists and engineers from nearly 200 institutions in 32 countries or regions. The observations were obtained in April 2017 and involved 19 observatories. In very-high-energy (VHE) gamma rays, this included observations by the High Energy Stereoscopic System (H.E.S.S.), the Major Atmospheric Gamma Imaging Cherenkov (MAGIC) telescopes, and the Very Energetic Radiation Imaging Telescope Array System (VERITAS). Collectively it resulted in the largest multi-wavelength campaign that includes the very-high-energy range on any active galaxy so far. The data of these 19 observatories is accessible at this webpage. All the data showed M87 in a quiescent state.
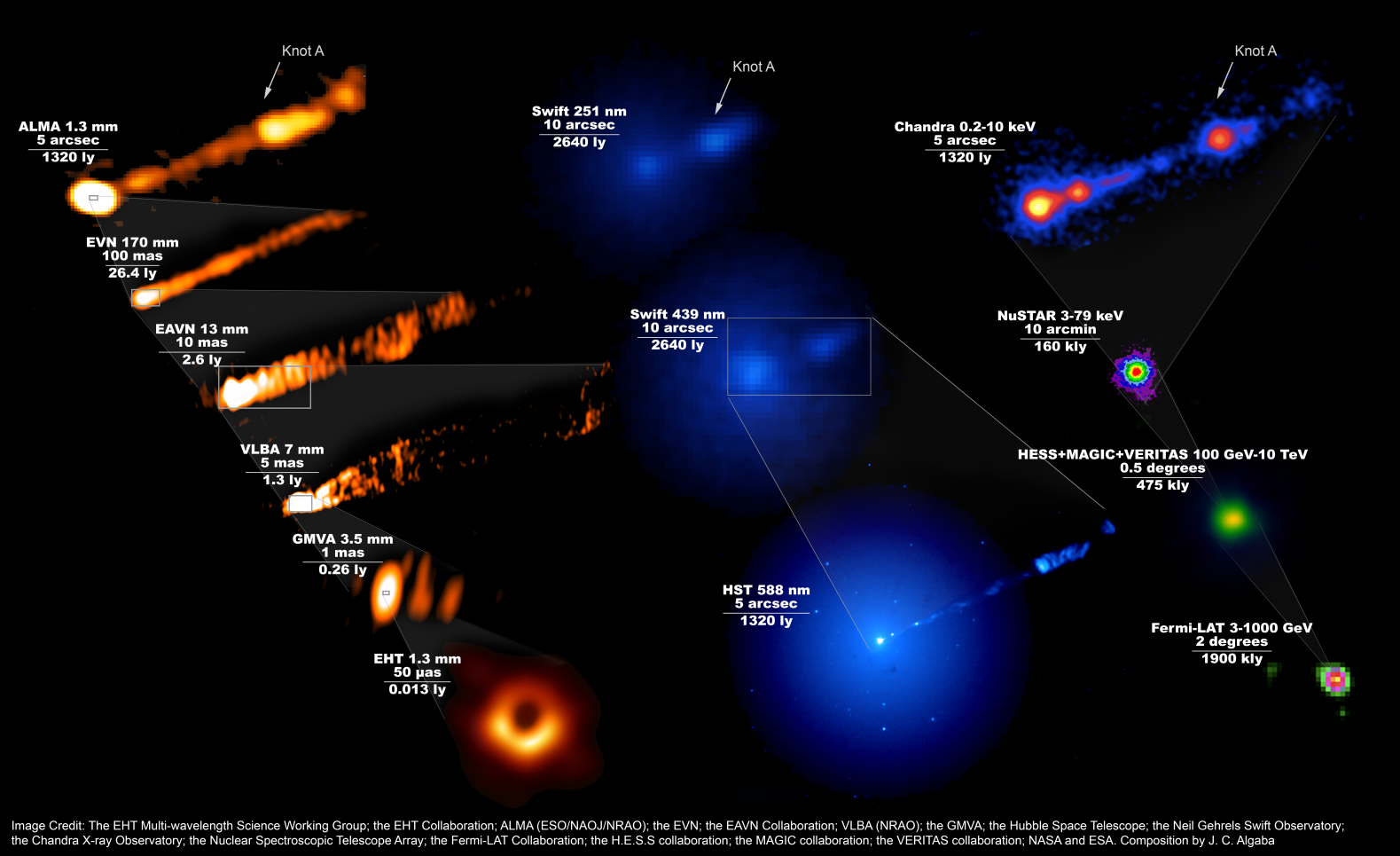
The distribution of the radiation measured by each instrument is shown in Figure 2. This 'spectral energy distribution' (SED) represents the total energy output in each wavelength band. The SED of a typical AGN shows twocomponents. Typically a maximum observed in the infrared, optical/UV or X-ray band, while a second one shows up at higher energies in the gamma-ray range. The low-energy component is well described by synchrotron radiation of electrons and positrons. These highly energetic electrons and positrons also scatter light and transfer their energy to the photons which thus become the gamma-rays that can be observed in the high-energy component. In many cases such models depend on many assumptions and do not offer very reliable predictions.
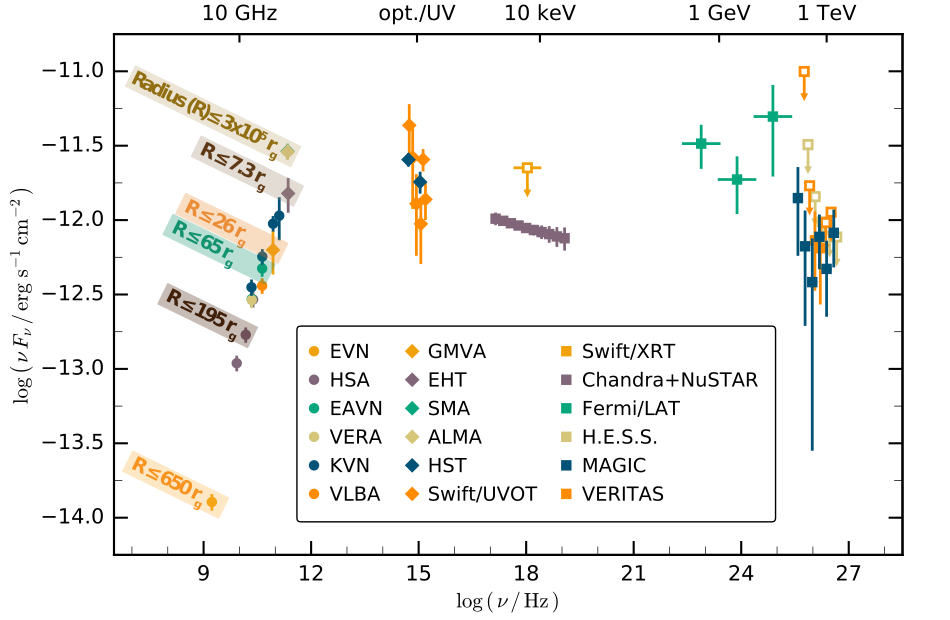
In the EHT campaign, however, it is possible to measure the size of the emission region as well as the magnetic field strength very close to the event horizon. These can be used to compute the expected scattered gamma-ray emission. Surprisingly, it turns out that the observed flux in the gamma-ray band is much higher than expected (Figure 3 left-hand diagram). An alternative model that are based on the observed gamma-ray emission is not able to explain the observed amount of radio emission (Figure 3, right-hand diagram) if conclusions obtained in earlier studies [3] and [4] are taken into account. The combined observations resulted in the best measurements of any AGN but also reveal that our understanding is still incomplete. Fortunately, more detailed models may be tested in correlated multi-wavelength variability in future campaigns. We will hopefully return to this question.
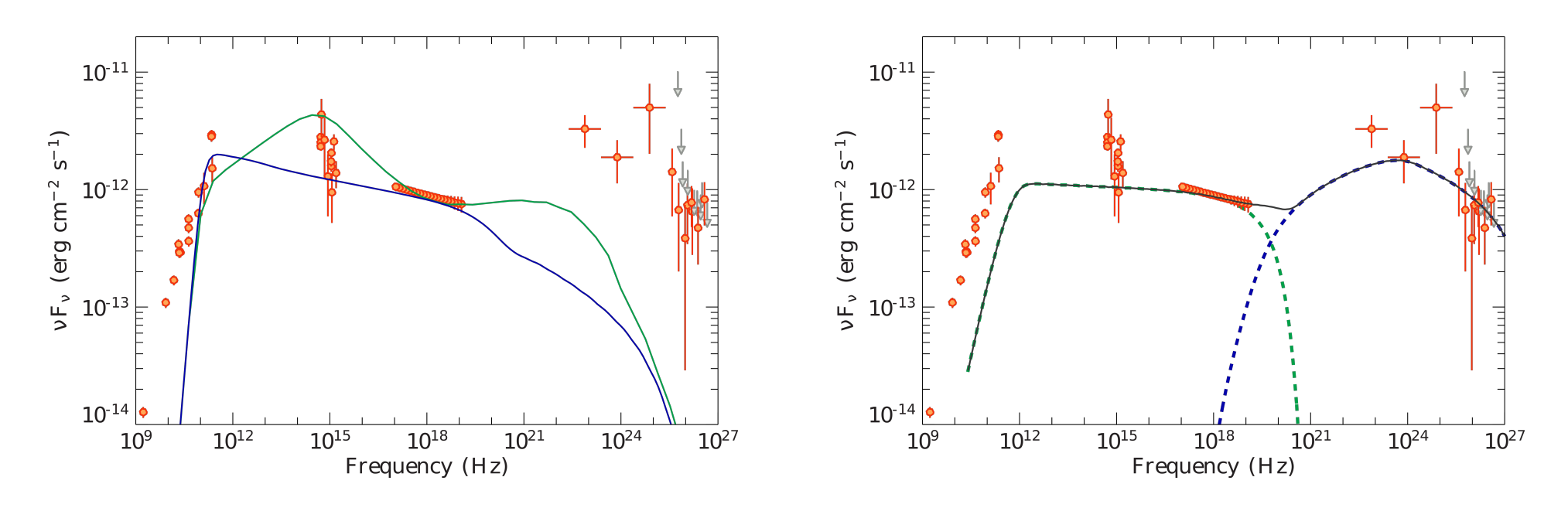
References:
[1] EHT Collaboration, et al., 2019a, First M87 Event Horizon Telescope Results. I. The Shadow of the Supermassive Black Hole, ApJL, 875, L1
[2] EHT MWL Science Working Group, et al., 2021, Broadband Multi-wavelength Properties of M87 during the 2017 Event Horizon Telescope Campaign, ApJL, 911, L11
[3] The VERITAS Collaboration, the VLBA 43 GHz M87 Monitoring Team, the H.E.S.S. Collaboration, the MAGIC Collaboration, et al., 2009, Radio Imaging of the Very-High-Energy γ-Ray Emission Region in the Central Engine of a Radio Galaxy, Science, 325, 444
[4] A. Abramowski et al., 2012, The 2010 Very High Energy γ-Ray Flare and 10 Years of Multi-wavelength Observations of M 87, ApJ, 746, 151