GW170817: Revisiting the site of a binary neutron star merger
October 2019
On August 17th 2017, the detection of the first electromagnetic counterpart to a gravitational-wave (GW) signal was achieved, originating from the binary neutron star (BNS) merger remnant. This result marked the start of multi-messenger astronomy. The event alone established the connection between short gamma-ray bursts and BNS mergers and it confirmed the forging of heavy elements in the so-called kilonova (see Figure 1 for a sketch). The H.E.S.S. telescopes were part of the most extensive world-wide campaign to search for an electromagnetic signal from the direction of the GW. These observations and their results are reported in [1] and are featured in our Source of the Month of November 2017.
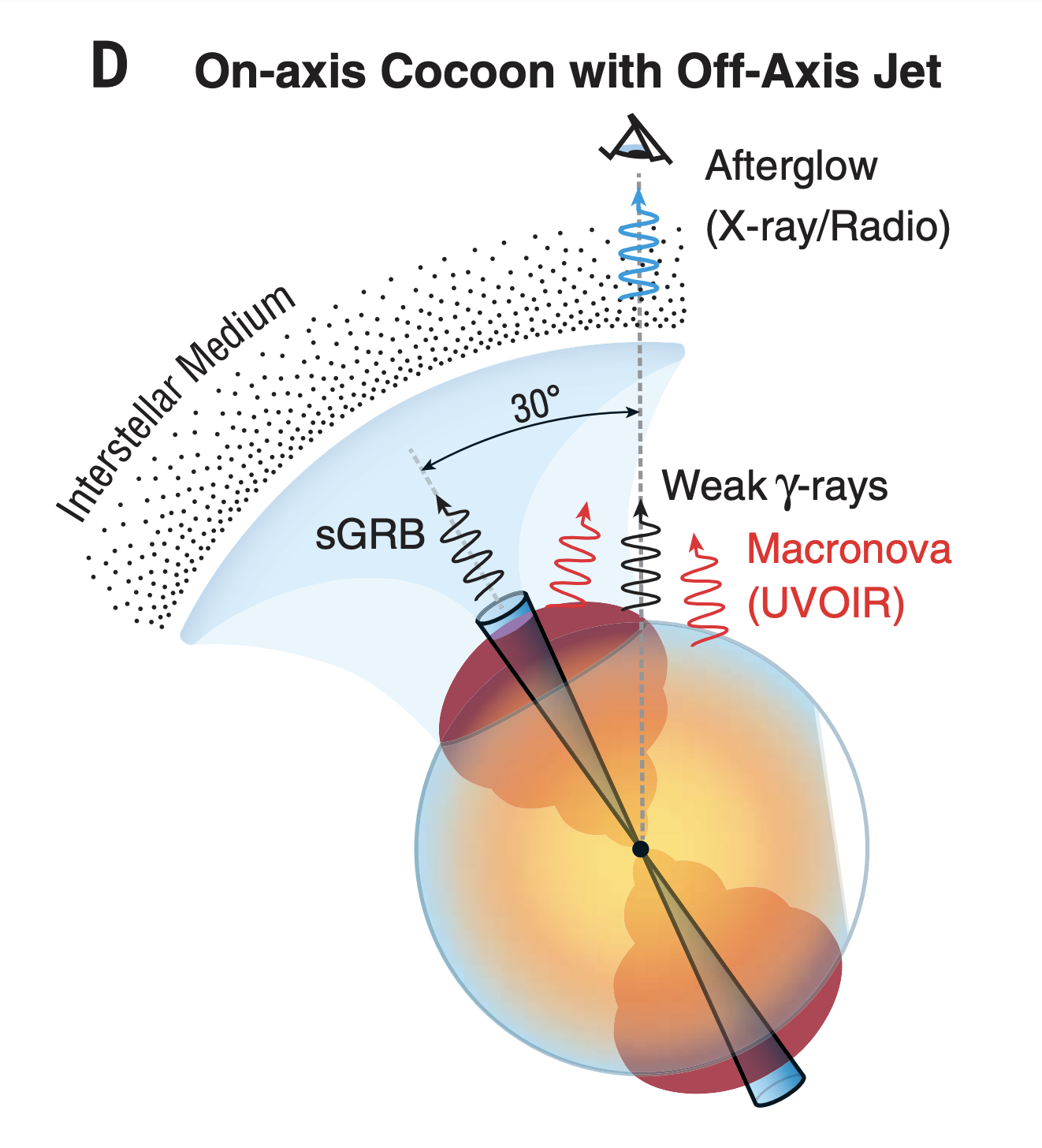
As material was propelled out of the system following the merger, and subsequently continued to expand and cool, the optical, ultraviolet and infrared emission kept dimming - so far so good....as expected. However, what surprised many astronomers was the fact that nine days after the merger, non-thermal emission, both in the radio and X-ray energy bands, was detected, and was observed to then brighten for almost the next half a year. Figure 2 illustrates this brightening, which was remarkably similar in both radio and X-rays. This may look like a conspiracy but is a clear signature of electrons being accelerated and emitting non-thermal photons when being deflected in magnetic fields - so-called synchrotron emission. H.E.S.S. covered the peak and plateau of this emission in observations conducted between December 2017 and May 2018.
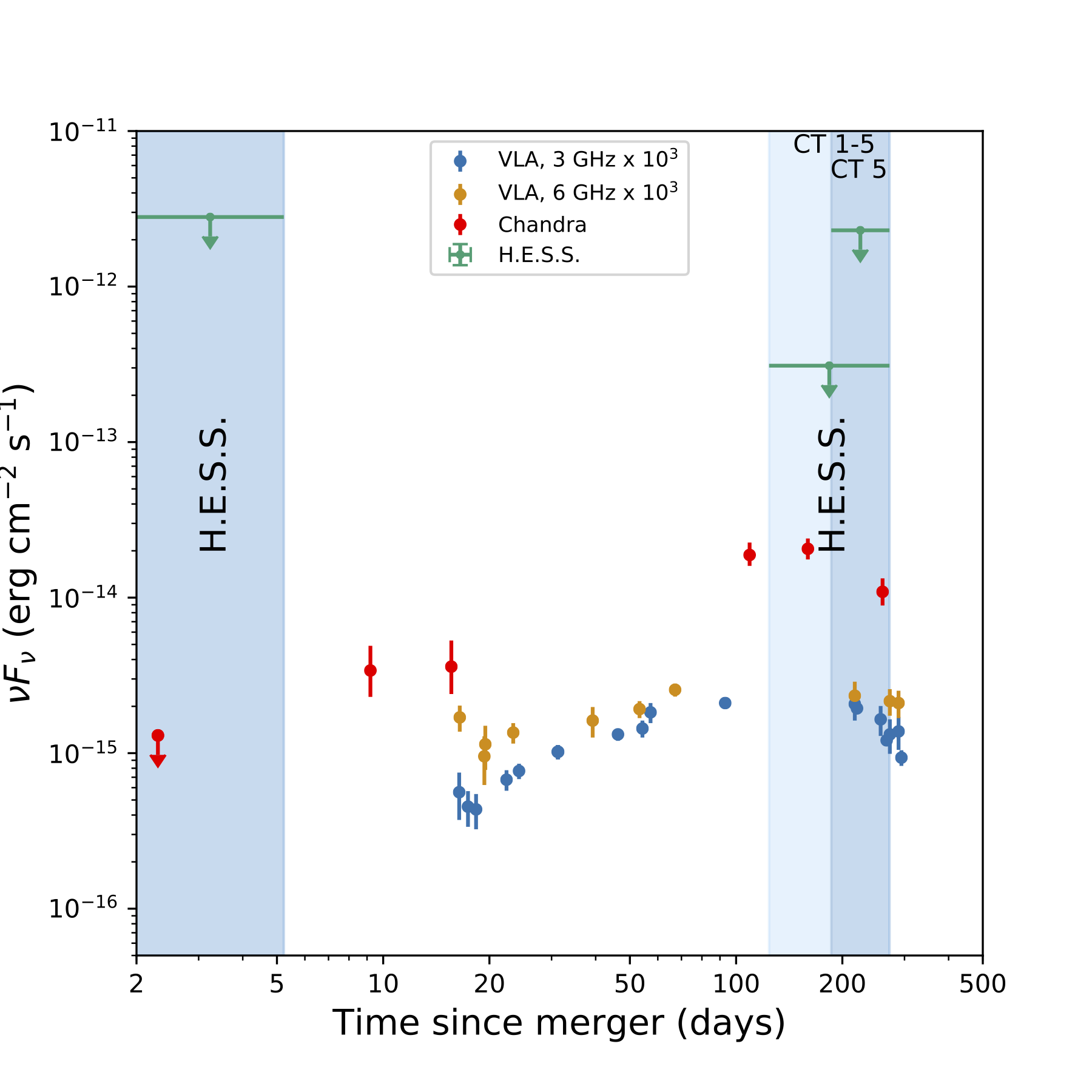
So why observe with H.E.S.S. at TeV energies, when the relativistic electrons emit synchrotron emission only to keV X-ray energies? The number of electrons are so abundant in the ejecta, that they will up-scatter the synchrotron photons they have just produced to much higher energies. Think of it as a football pitch filled with players, one player kicks the ball, while another one gives the ball another kick making it even faster. This emission mechanism is well established in objects like the Crab Pulsar Wind Nebula. But what is the expected emission level in the H.E.S.S. domain? Well, this depends on the number of electrons as well as the magnetic field strength... and it is notoriously hard to measure magnetic fields in astrophysical sources. The higher the magnetic field strength, the fewer the number of electrons that are required to explain the measured synchrotron emission. On the other hand, if magnetic fields are low, more electrons are required to produce the measured radio and X-rays. The problem is that by measuring the synchrotron emission alone, we cannot disentangle the two contributors. This is where imaging atmospheric Cherenkov telescopes come in and can break the ambiguity. Different models have been developed before and after the discovery of non-thermal emission following BNS mergers, which try to explain and predict the synchrotron as well as self-Compton emission (e.g. [3,4]).
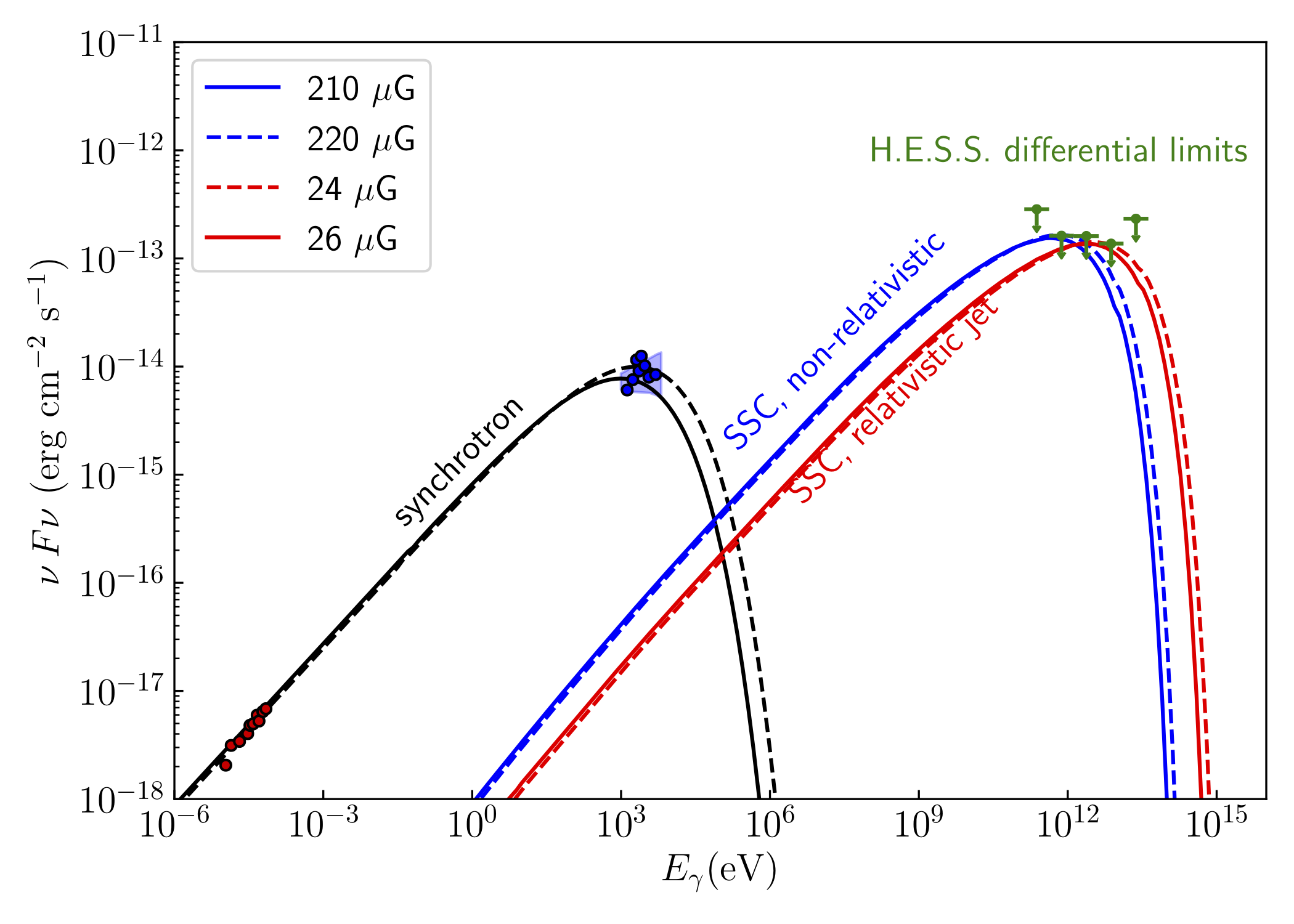
For quite some time, there has been a debate within the community if the non-thermal emission originated from the kilonova-like outflow, or from the relativistic off-axis jet. It was the detection of superluminal motion in radio wavelengths, which confirmed that indeed the jet that was launched in the BNS merger is at least one of the sites in the source where acceleration takes place [5]. We took a deep look at the region of the GW170817 event for more than 100 days to search for the respective self-Compton emission. However we unfortunately didn't detect a signal. Nevertheless, these deep observation and non-detection provide strong upper limits, which can in combination with radio and X-ray emission be translated into limits on the minimum magnetic field in the merger remnant. We have derived these limits and translated them into limits on the magnetic field in the two scenarios: 1) for the case that the spherically expanding kilonova is responsible for the emission, and 2) that the emission indeed originates from the jet seen off-axis. The results we found are shown in Figure 2 and illustrate that magnetic fields of at least ~20 micro-Gauss in the jet scenario, or 200 micro-Gauss in the kilonova scenario are required to explain the synchrotron emission and not violate the H.E.S.S. measurement (Figure 3).
H.E.S.S. article: "Probing the magnetic field in the GW170817 outflow using H.E.S.S. observations", H.E.S.S. Collaboration (in internal review)
References:
[1] H.E.S.S. Collaboration (H. Abdalla et al.), "TeV gamma-ray observations of the binary neutron star merger GW170817 with H.E.S.S.", Astrophys. J. Lett 850 (2017) 2
[2] M.M. Kasliwal et al., "Illuminating gravitational waves: A concordant picture of photons from a neutron star merger", Science 358, 1559-1565
[3] H. Takami, K. Kyutoku, and K. Ioka, "High-energy radiation from remnants of neutron star binary mergers", Phys. Rev. D 89 (2014) 063006
[4] X. Rodrigues, D. Biehl, D. Boncioli, and A. M. Taylor, "Binary neutron star merger remnants as sources of cosmic rays below the 'Ankle'", Astropart. Phys. 106 (2019) 10
[5] K. P. Mooley et el., "Superluminal motion of a relativistic jet in the neutron-star merger GW170817", Nature 561 (2018) 355