An active proton PeVatron hiding inside HESS J1702-420?
August 2021
More than a century after their discovery in 1912 [1], the origin of cosmic rays remains one of the oldest unsolved mysteries in physics. The bulk of cosmic rays reaching the Earth — mostly energetic protons — originate within our Galaxy, at unknown sites where powerful objects called PeVatrons accelerate them at least up to PeV (1 PeV = 1015 eV) energies. To date we still lack an unambiguous observational proof that any specific Galactic source operates as a proton PeVatron. In a new study [2], the mystery object HESS J1702-420 has now been identified as an excellent PeVatron candidate - 15 years after its discovery [3]. This source still challenges our understanding of the high-energy Universe - mostly because it is a dark TeV source, which means that it has been observed only in the TeV (1 TeV = 1012 eV) γ-ray band while it completely disappears at lower energies. Soon after its discovery, a dedicated study revealed that HESS J1702-420 has a hard γ-ray power law spectrum, which is compatible with the expectation for Galactic PeVatrons [4]. Further observations of HESS J1702-420 with the H.E.S.S. updated cameras provided exciting new results.
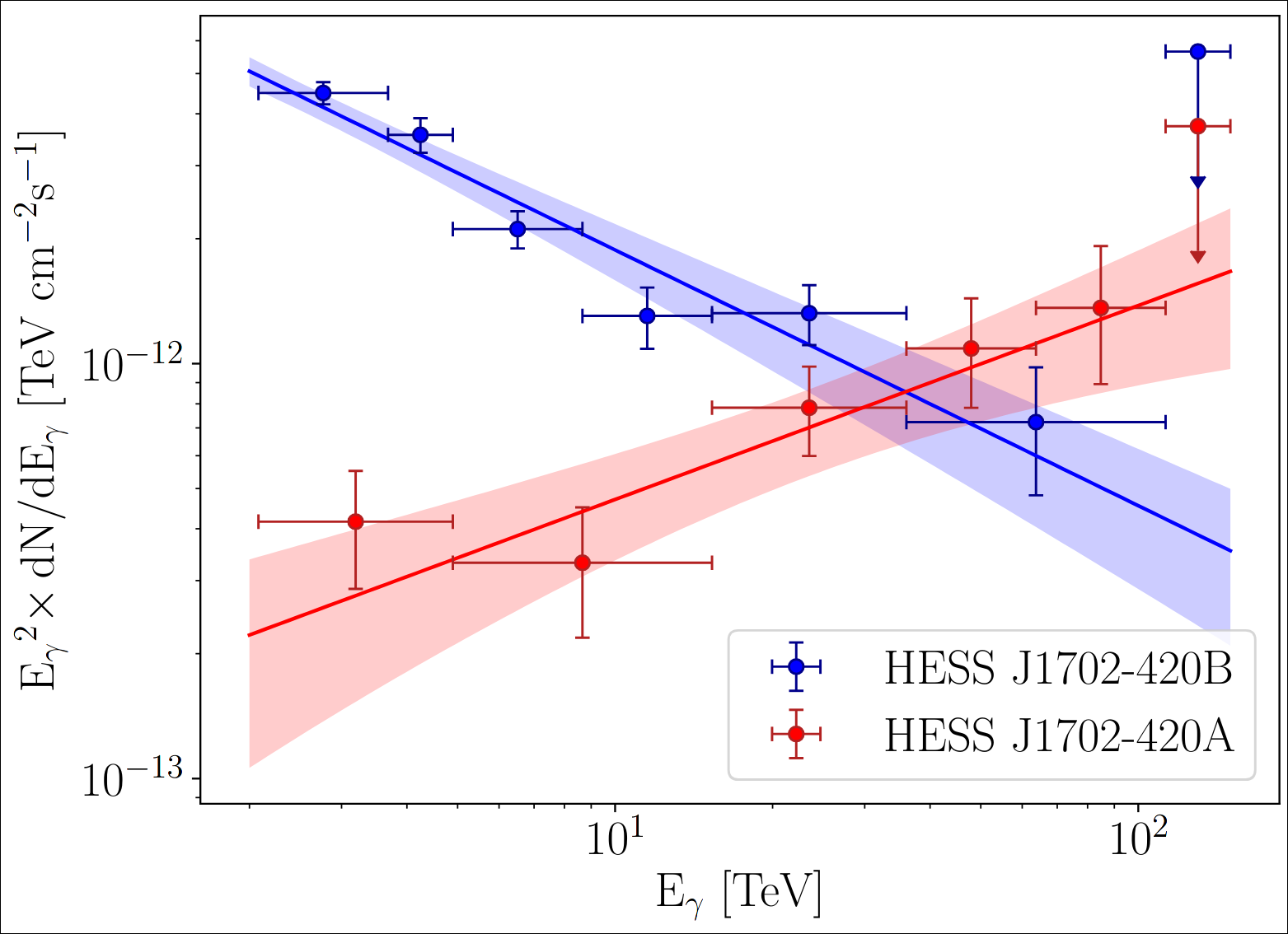
For the first time, H.E.S.S. observations have been processed with an open source high-level analysis tool called gammapy [5]. This allowed us to adopt a new analysis approach, known as 3D modeling. This technique essentially consists in the adjustment of a three dimensional (two angular coordinates and energy) model to the data, in such a way that the model-predicted count map matches as closely as possible with the measured one. This way, we could separate two sources inside HESS J1702-420 based on their different morphologies and γ-ray spectra. One of the two components, named HESS J1702-420A, has a remarkably hard power law spectral index (Γ = 1.53 ± 0.19stat ± 0.20sys) and was detected by H.E.S.S. at a 4.0σ confidence level in the energy band 64 − 113 TeV. This is an unprecedented achievement for the H.E.S.S. experiment and brings evidence for the source emission up to 100 TeV. Below a few tens of TeV, HESS J1702-420A is outshone by the other component, HESS J1702-420B, which has a steep spectral index of Γ ≈ 2.6, an elongated shape and accounts for most of the low-energy HESS J1702-420 emission. Figure 1 shows the spectra of both sources. Figure 2 instead presents energy-dependent γ-ray flux maps, which illustrate the transition between a low energy regime — dominated by the steep spectrum of HESS J1702-420B — to a high energy one, in which HESS J1702-420A stands out with its exceptionally hard power law spectrum. We notice that Figure 2 suggests that the γ-ray emission converges towards the position of the unidentified X-ray source Suzaku source B with increasing energy.
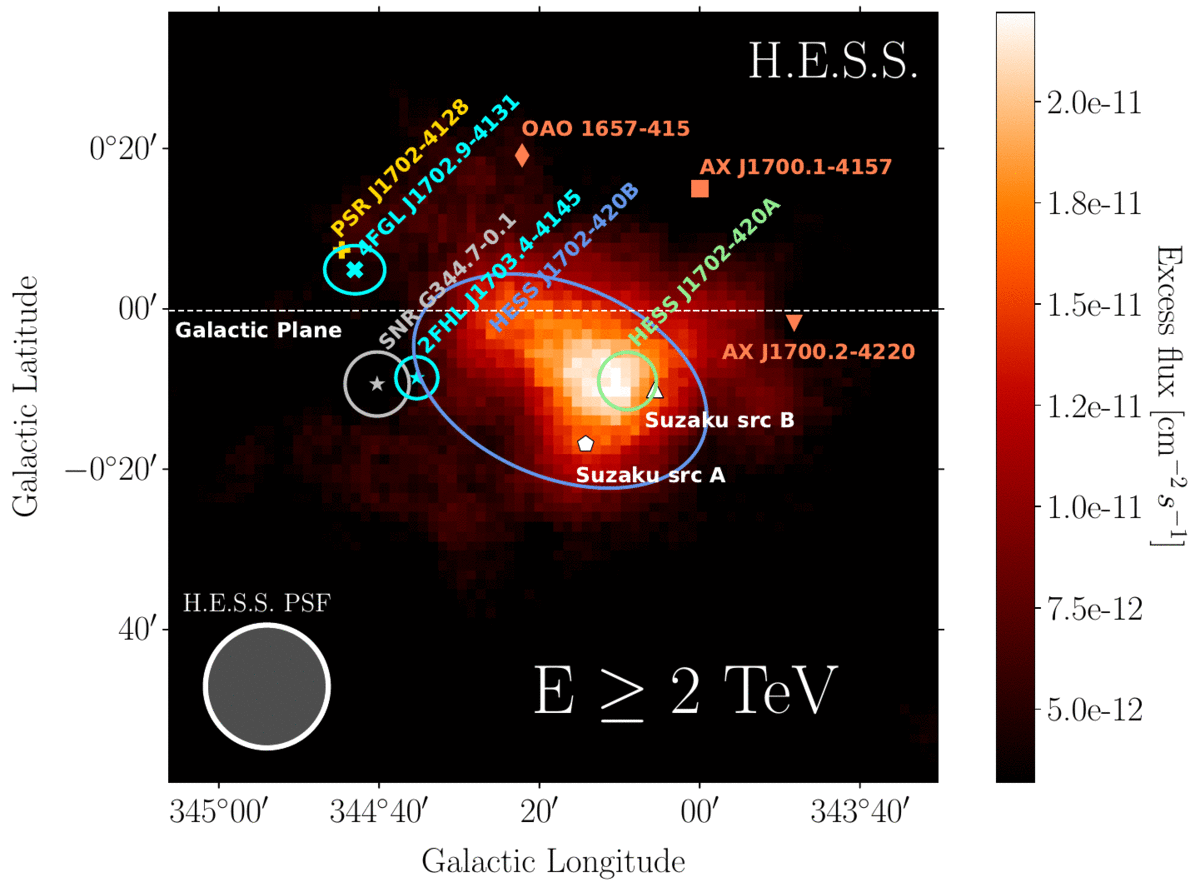
The discovery of HESS J1702-420A opens up the possibility that a HESS J1702-420 harbors an active proton PeVatron. Therefore we used physically-motivated non-thermal radiative models from the naima code [6] to explore simple one-zone hadronic (based on p-p interactions and subsequent π0 decay) and leptonic (based on the inverse Compton process) emission scenarios. We found that a pure power law distribution of protons (electrons) with slope Γp ≈ 1.58 (Γe ≈ 1.61) is well suited to explain the γ-ray emission from HESS J1702-420A. Figure 3 shows a comparison of the two models, from which it is clear that at the moment neither of the two scenarios can be discarded.
Remarkably, in a hadronic scenario, the cut-off energy of the proton distribution powering HESS J1702-420A is found to be higher than 500 TeV at a 95% confidence level. For such a scenario, this implies that the source likely harbors protons up to PeV energies, which makes it the most compelling PeVatron candidates detected in H.E.S.S. data so far.
However, we notice that a proton spectrum with such a hard slope over two decades is in tension with the standard prediction from diffusive shock acceleration (Γ ≈ 2, [7]). This fact may suggest that HESS J1702-420A, instead of being a proton accelerator, is in fact a gas cloud that is illuminated by cosmic rays transported from elsewhere. In that case, the hard measured proton spectrum could be explained by the escape of the most energetic particles from a nearby proton PeVatron [8]. Alternatively, the γ-ray emission from HESS J1702-420A might be interpreted as the hard high energy end of a concave spectrum arising from nonlinear shock acceleration [9], or originate from the interaction of supernova remnant shock waves with a young stellar cluster wind [10]. The absence of a clear spatial correlation between the interstellar gas distribution and the observed TeV emission [11] prevents however a confirmation of the hadronic scenario, unless an extremely powerful hidden PeVatron is present. In the latter case, even a modest gas density would suffice to produce the measured γ-ray emission of HESS J1702-420A, explaining the observed nonlinearity between the interstellar medium and TeV maps.
A leptonic emission scenario, which would potentially dismiss the PeVatron hypothesis for HESS J1702-420, could not be ruled out. In particular, the proximity of HESS J1702-420A with the unidentified Suzaku source B might point toward a pulsar wind nebula scenario. Such association is however challenged by the absence of a synchrotron cooling break in the spectrum of HESS J1702-420A, and by the extremely low magnetic field that it would imply (B ≈ 0.3 μG). We notice that an alternative interpretation is possible, in which the observed emission from HESS J1702-420 is due to electrons that are accelerated by the reconnection electric field at X-points in the current sheets of a pulsar striped wind, where the magnetic field value is expected to be low [12]. If true, this would be the first time that a TeV measurement probes the reconnection spectrum immediately downstream of the termination shock of a pulsar wind.
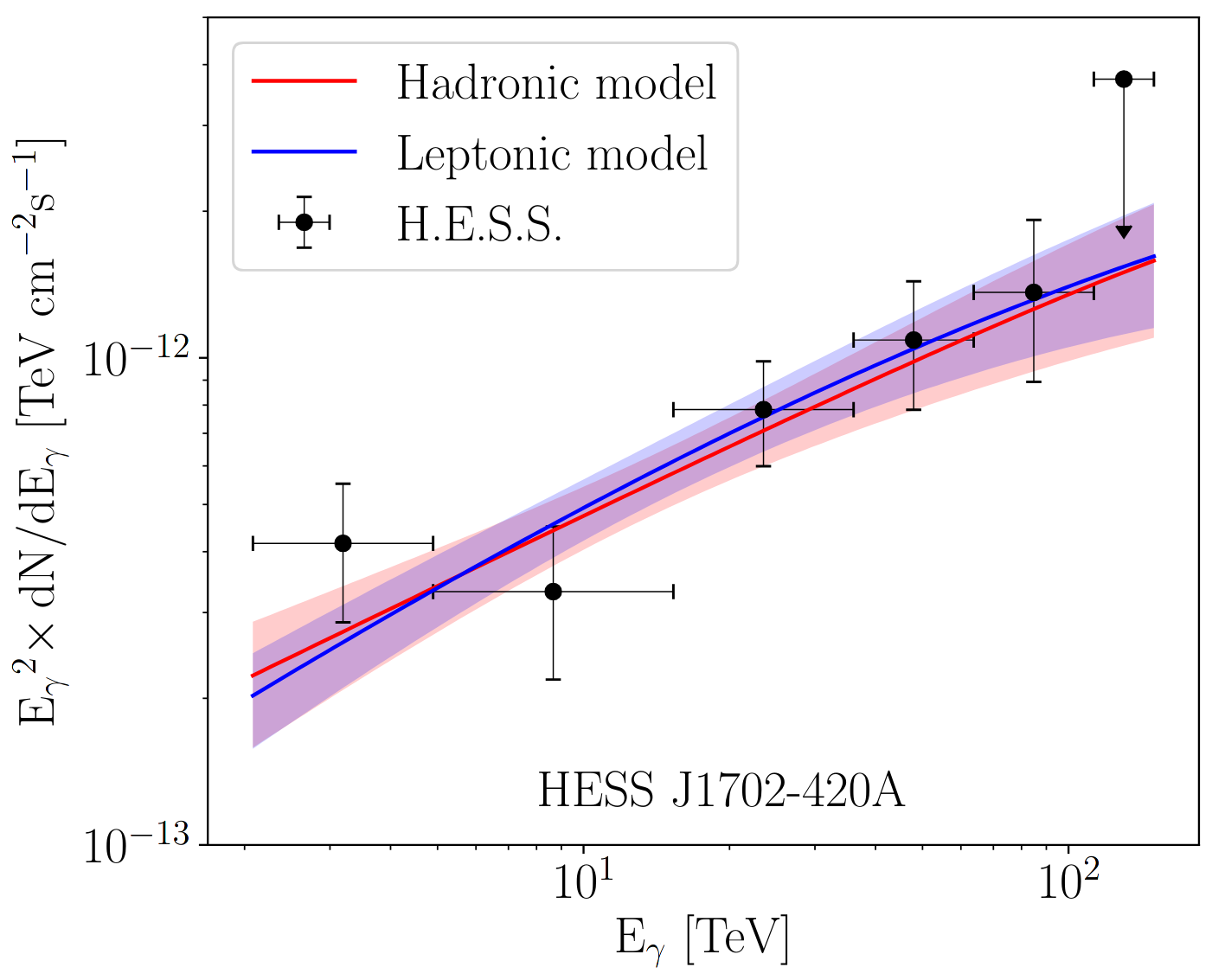
Further observations at high energies will possibly close the debate on the nature of HESS J1702-420. In particular, deep measurements at energies >100 TeV will constrain the spectral shape of HESS J1702-420A near the cut-off region, thus probing its hadronic or leptonic origin and determining whether it is compatible with the presence of a real cosmic ray PeVatron. Finally, observations in the X-ray band will also be important to search for a multi wavelength counterpart of the TeV source, and clarify the relationship between HESS J1702-420A and the unidentified Suzaku source B.
[1] Hess, V., “On the Observations of the Penetrating Radiation during Seven Balloon Flights”, Physikalische Zeitschrift 13 (1912) 1084
[2] H.E.S.S. collaboration, Abdalla, H., et al.,“Evidence of 100 TeV γ-ray emission from HESS J1702-420: A new PeVatron candidate”, Accepted for publication in Astronomy & Astrophysics, 10.1051/0004-6361/202140962
[3] H.E.S.S. collaboration, Aharonian, F., “The H.E.S.S. Survey of the Inner Galaxy in Very High Energy Gamma Rays”, The Astrophysical Journal, vol. 636, no. 2, pp. 777–797, 2006. doi:10.1086/498013.
[4] H.E.S.S. collaboration, Aharonian, F., “HESS very-high-energy gamma-ray sources without identified counterparts”, Astronomy and Astrophysics, vol. 477, no. 1, pp. 353–363, 2008. doi:10.1051/0004-6361:20078516.
[5] Deil, C., Donath, A., Terrier, R., et al. 2020, gammapy/gammapy: v0.17, 10.5281/zenodo.4701492
[6] Zabalza, V. 2015, Proc. of International Cosmic Ray Conference 2015, 922
[7] Bell, A.R., The Acceleration of cosmic rays in shock fronts. I, Mon. Not. Roy. Astron. Soc. 182 (1978) 147.
[8] Gabici, S., Aharonian, F.A. and Casanova, S.: Broad-band non-thermal emission from molecular clouds illuminated by cosmic rays from nearby supernova remnants, Monthly Notices of the Royal Astronomical Society, 396 (2009) 1629 [0901.4549].
[9] Kang, H., Ryu, D. and Jones, T.W., Self-Similar Evolution of Cosmic-Ray Modified Shocks: The Cosmic-Ray Spectrum, 695 (2009) 1273 [0901.1702].
[10] Bykov, A.M. et al., Ultrahard spectra of PeV neutrinos from supernovae in compact star clusters, Monthly Notices of the Royal Astronomical Society 453 (2015) 113.
[11] Lau, J.C. et al., Probing the origin of the unidentified TeV -ray source HESSJ1702–420 via the surrounding interstellar medium, Monthly Notices of the Royal Astronomical Society 483 (2018) 3659
[12] Sironi, L. and Spitkovsky, A., Acceleration of particles at the termination shock of a relativistic striped wind, The Astrophysical Journal 741 (2011) 39.