GRB 190829A: A long-lasting explosion offers surprises
June 2021
Gamma-ray bursts (GRBs) are bright X-ray and gamma-ray flashes observed in the sky, emitted by distant extragalactic sources. They are associated with the collapse of rapidly rotating massive stars, in which a fraction of the liberated gravitational energy feeds the production of blast wave moving close to the speed of light. Their emission is divided into two distinct phases: an initial spiky prompt phase lasting tens of seconds, followed by a long-lasting, smoothly fading afterglow phase, detectable for a long period after the burst. This X-ray afterglow radiation is emitted by accelerated electrons which interact and lose energy with the blast wave magnetic field. This energy lost is radiated in the form of synchrotron photons. Due to the simplicity of the underlying afterglow physics, they are considered as an excellent cosmic laboratory for the study of relativistic shocks. Although the synchrotron emission process that produces the X-ray emission is well understood, it has remained unclear whether a very high energy (VHE, >100 GeV) gamma-ray component exists, and if it does, what the emission process giving rise to this component is.
The detection of GRBs in the VHE gamma-ray regime has been a missing piece of the multiwavelength puzzle. The detection of VHE emission is particularly difficult, as the distant Universe becomes non-transparent to multi-TeV gamma rays due to their absorption with background light that permeates the Universe (see Figure 1). The detection of GRBs in this regime has been a focus of gamma-ray astronomers, and major efforts have been made to improve the performance and follow-up strategies of the telescopes. This was rewarded recently by the detection of two GRBs at VHE energies: the first 10 hours after the afterglow onset [1], a second one within the first hour of the afterglow [2]. These detections revealed that bright VHE afterglow emission is indeed produced and is observable for at least half a day after the initial event; however, these particular GRBs were each observed for less than two hours, and the origin and evolution of the VHE emission remained inconclusive. In addition, these GRBs were at moderate distances, so their spectrum above 1 TeV could not be probed. Recently, an international team of researchers with the H.E.S.S. (High Energy Stereoscopic System) array of atmospheric Cherenkov telescopes, reported the detection of a third GRB — at a redshift of only z=0.0785, a mere 1 billion light-years away! [3].
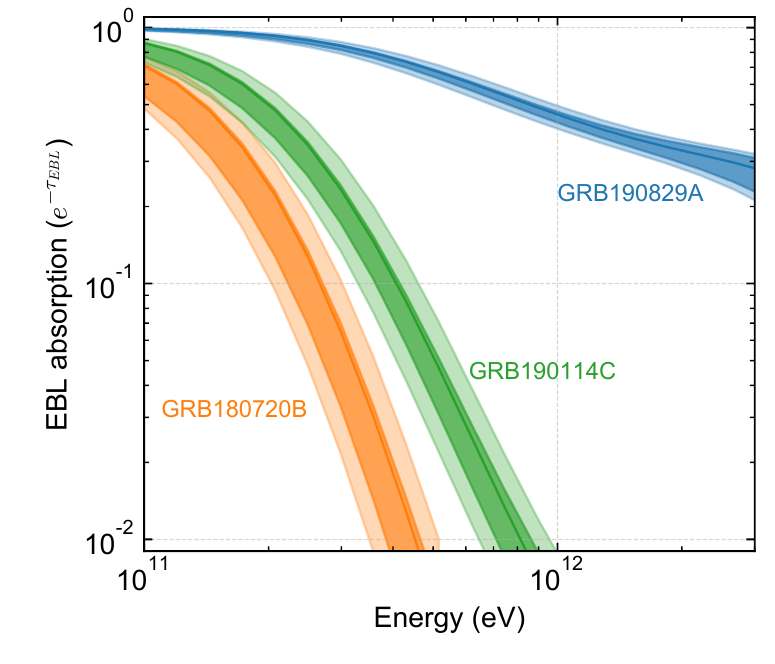
On 29 August 2019, the Fermi Gamma-Ray Burst Monitor and the Swift Burst Alert Telescope detected and localised GRB 190829A. Subsequently, ground-based observatories including H.E.S.S. turned to look at this position to obtain good time coverage of this event over a wide range of energies. Observations with H.E.S.S. started 4 hours after the burst, when the source first became visible to its telescopes, resulting in the detection of VHE emission which was quickly telegramed to the astronomical community. H.E.S.S. continued to observe and detect the GRB190829A for several days. The data obtained by H.E.S.S. has lead to two new unique observational insights about GRB afterglows. The first of these is the detection of a VHE GRB light-curve over multiple days, from 4 to 56 hours after the burst (see Figure 2). The second achievement is the accurate determination of the spectrum over more than an order of magnitude in energy and reaching unprecedented energies, from 0.18 to 3.3 TeV. These observational landmarks were accomplished by a combination of the good sensitivity of the instrument, and the fortuitous proximity of the GRB. The accurate measurements over a broad energy range have allowed the intrinsic VHE spectrum to be reliably probed for the first time.
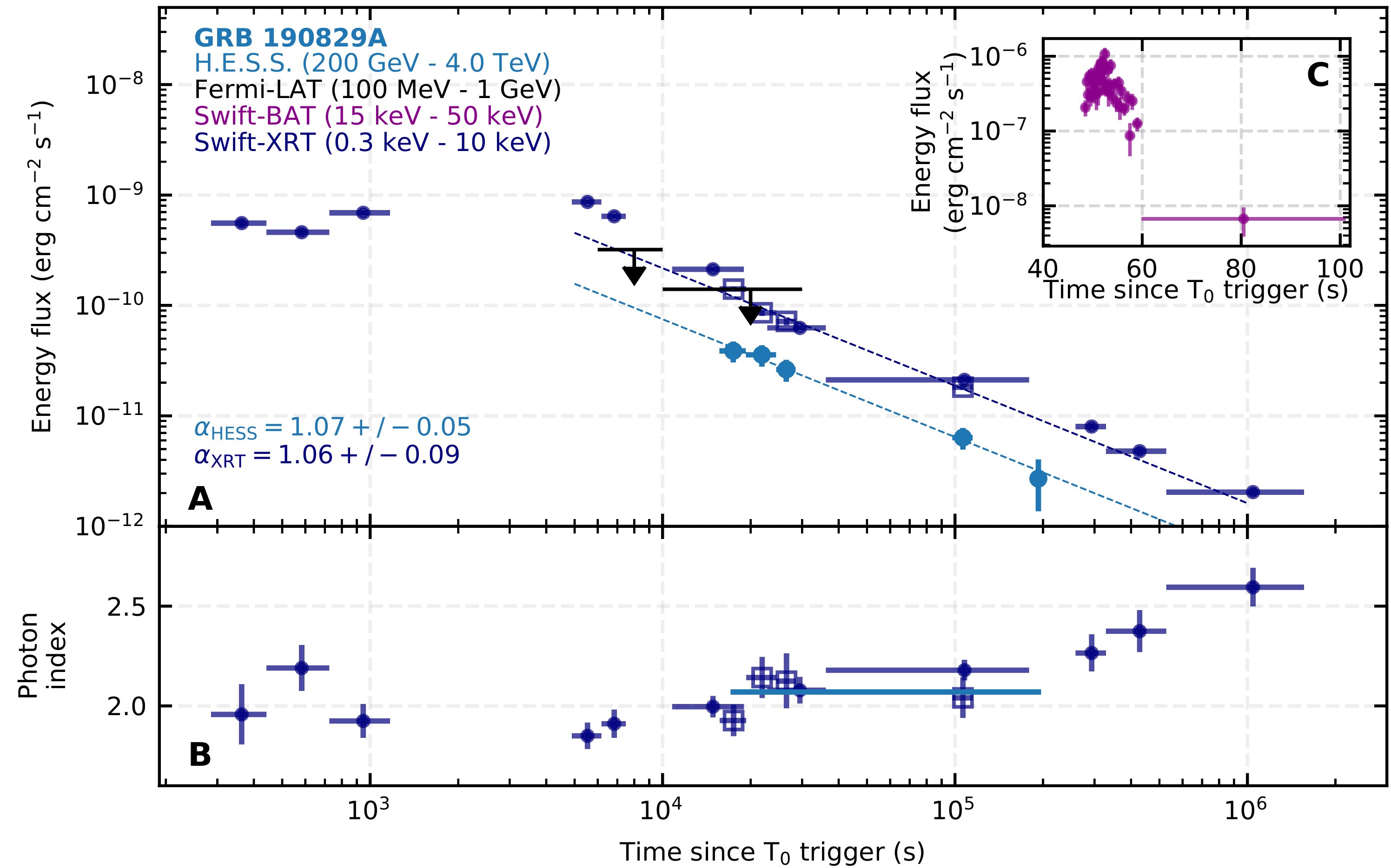
This new result has revealed curious similarities between the X-ray and VHE gamma-ray emission. The intrinsic spectrum of the GRB measured by HESS smoothly matches a continuation of the X-ray spectrum up to much higher energies. Moreover, the X-ray and VHE gamma-ray emission dim in a strikingly similar way (see Figure 2). It is rather unexpected to observe such remarkably similar spectral and temporal characteristics in the X-ray and VHE gamma-ray energy bands, if the emission in these two energy ranges have different origins. This result therefore poses a challenge for a typically invoked synchrotron self-Compton (SSC) origin of the VHE emission, in which the accelerated electrons boost the synchrotron photons to higher energies. Interestingly, the H.E.S.S. observational result does fit into a simple framework in which, instead, synchrotron emission is responsible for both the X-ray and VHE gamma-ray emission (see Figure 3). Such a result, however, would require that accelerated electrons have energies well beyond the burn-off limit, set by the balance of acceleration and energy loss rates within the accelerator. The far-reaching implication of this possibility highlights the need for further studies of VHE GRB afterglow emission.
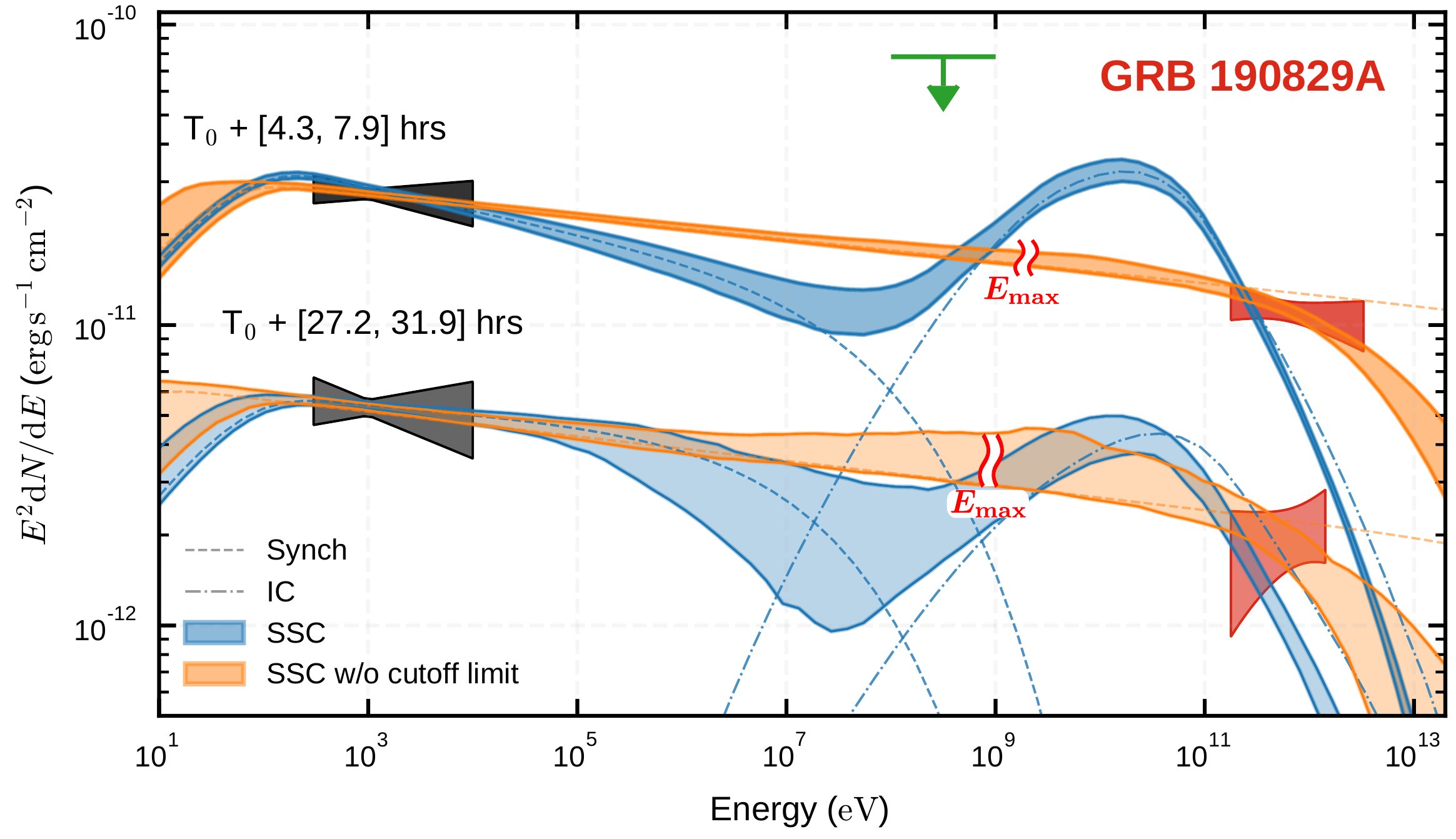
Looking to the future, the prospects for the detection of GRBs by next-generation instruments look promising. Certainly, the abundance of GRB detections over the last few years leads astronomers to expect that the experience gained may result in detections in the VHE band becoming more common. However, a high bar has now been set by this HESS result, which has highlighted the scientific importance for the detection at VHE of local GRBs, particularly at late times in the afterglow.
References:
[1] H.E.S.S. Collaboration (Abdalla, H. et al.), 2019, A very-high-energy component deep in the γ-ray burst afterglow, Nature, 575, 464
[2] , Acciari, V.A., et al. (MAGIC collaboration), 2019, Teraelectronvolt emission from the γ-ray burst GRB 190114C, Nature, 575, 455
[3] H.E.S.S. Collaboration, 2021, Revealing x-ray and gamma ray temporal and spectral similarities in the GRB 190829A afterglow, Science, 372, 1081