One Year Later - LS 5039 Revisited
August 2006
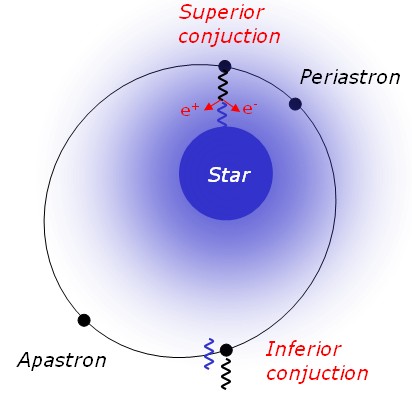
One year ago, in August 2005, the microquasar LS 5039 (top figure) was featured as source of the month. Novel exciting results on this source merit a renewed discussion, despite the many other H.E.S.S. sources which await presentation. LS 5039 was the first microquasar established as a high-energy gamma-ray source (Aharonian et al. 2005), more recently followed by LS I +61 303 (Albert et al. 2006) as a second example. Such binary systems with eccentric orbits provide a unique laboratory to study how particle acceleration and propagation vary with the changing environment along the orbit. Orbital X-ray variability of LS 5039 was indeed reported byBosch-Ramon et al. (2005). Variation of high-energy gamma-ray emission from binaries has been observed on sections of the 3.4-year orbit of the binary pulsar PSR B1259-63/SS 2883 by H.E.S.S. (April 2005) and along the 26 day orbit of LS I +61 303 by MAGIC (Albert et al. 2006). With its 3.9 day orbit, LS 5039 now allows for the first time recording many periods and establishing periodic variation of the gamma-ray flux along the orbit.
In 2004 and 2005, H.E.S.S. accumulated 69 hours of data on LS 5039, boosting the gamma-ray signal to 40 standard deviations above background. In the 160 observation runs, significant variability of the gamma-ray emission was immediately obvious. A periodicity analysis using the Lomb-Scargle test (Scargle 1982)- similar to a Fourier decomposition of the time-variable flux into its frequency components - shows a strong frequency component at a period of 3.908 days (Fig. 1), within errors consistent with the know orbital period of 3.906 days (Casares et al. 2005). The nearby source HESS J1825-137, observed simultaneously, showed no modulation, excluding any kind of instrumental artifact. Folding the measured light curve with the period results in Fig. 2, showing gamma-ray flux as a function of orbital phase. Orbital modulation is clearly visible. However, contrary to naive expectations, the maximum emission is not at periastron, but rather around the inferior conjunction, where the compact object and the star line up along the line of sight. Periastron - the closest approach - should provide the highest accretion rate onto the compact object, and the highest magnetic and photon fields, which should boost particle acceleration and gamma-ray production (unless radiative energy losses are so high that they prevent further acceleration). However, if gamma rays are created close to the compact object, then another effect becomes important: absorption of gamma rays in collisions with stellar photons (e.g.Böttcher and Dermer 2005,Dubus 2006,Bednarek 2006). When the compact object is "behind" the star, gamma rays and stellar photons collide head-on and can easily surpass the threshold for electron-positron pair production, resulting in a nearly 100% loss of high-energy photons (top figure; stellar photons are shown in blue and high-energy gamma rays in black). At the inferior conjunction, when the compact object is "in front", gamma rays and stellar photons travel on parallel paths and cannot interact, therefore the flux maximum. However, this cannot be the full story. The modulation of the gamma-ray spectrum is strongly energy-dependent (Fig. 3): around 200 GeV and 30 TeV, the flux does not vary strongly with orbital phase, whereas at a few TeV, variation is as large as a factor 5. Absorption alone, however, should generate a significant modulation already at 200 GeV. It seems that - not quite unexpectedly - orbital variation of the particle acceleration partly compensates for the absorption and results in a complex, energy-dependent flux modulation. Recent articles which discuss gamma-ray production mechanism and give further references are e.g.Bosch-Ramon et al. (2006),Paredes et al. (2006),Gupta et al. (2006) andDermer and Böttcher (2006). Besides the leptonic origin discussed in these papers, also hadron acceleration has been considered (e.g. Aharonian et al. 2006, in press, Bosch-Ramon et al. 2006)
References:
3.9 day orbital modulation in the TeV gamma-ray flux and spectrum from the X-ray binary LS 5039, H.E.S.S. collaboration, F. Aharonian et al., astro-ph/0607192 (2006).
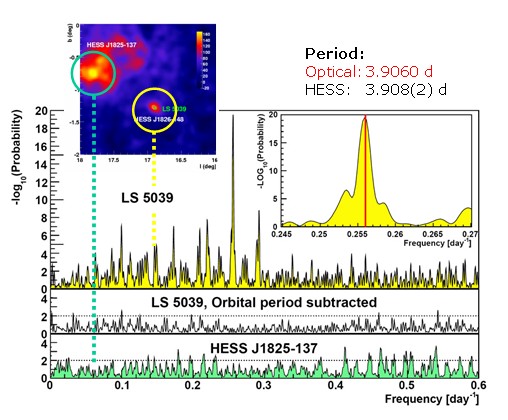
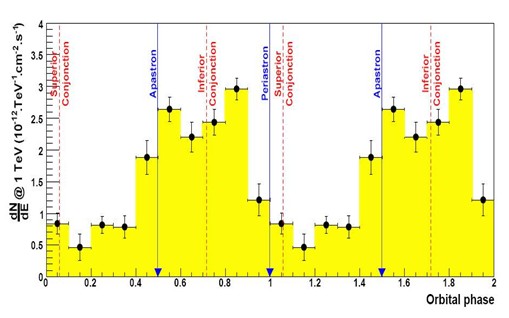