Caught in the Act: AGN flares are everywhere
February 2022
When we observe the sky in gamma rays of the highest energies, most of the sources outside of our Milky Way turn out to be the central regions of other galaxies. These active galactic nuclei (AGN) are found to be in only a few percent of all galaxies and they are among the most luminous objects in the Universe. An AGN is powered by the black hole in the center of its host galaxy that is millions or even billions of times more massive than the Sun [1]. These black holes swallow the surrounding material and the radiation released in the process may outshine the light from the billions of stars in the entire host galaxy. Some AGN eject energetic outflows – called 'jets' – which we can observe with telescopes across all wavelengths. They can often show fascinating behaviour by changing their brightness in short periods of time which can range from years, to months, down to just a few minutes in gamma rays (H.E.S.S. SOM Jul. 2017, SOM Jan. 2020, SOM Mar. 2020, Fig. 1). These ultra-fast variations can tell us about the physical processes happening in the vicinity of black holes, like how the participating particles can reach speeds as fast as the the speed of light and how the subsequent radiation arriving at Earth – from radio waves all the way to gamma rays – is produced. These dramatic flaring episodes of AGN seem to appear spontaneously and are therefore impossible to predict. The observation of such events requires a very fast reaction time across the entire electromagnetic spectrum, ideally with coordinated, simultaneous observations in multiple bands. Such coordinated observations, triggered by the detection of an AGN flare in one waveband, are termed Target of Opportunity (ToO) observations. Over the past years, observational strategies for such ToO observations have significantly improved, leading to deeper insights into the violent Universe.
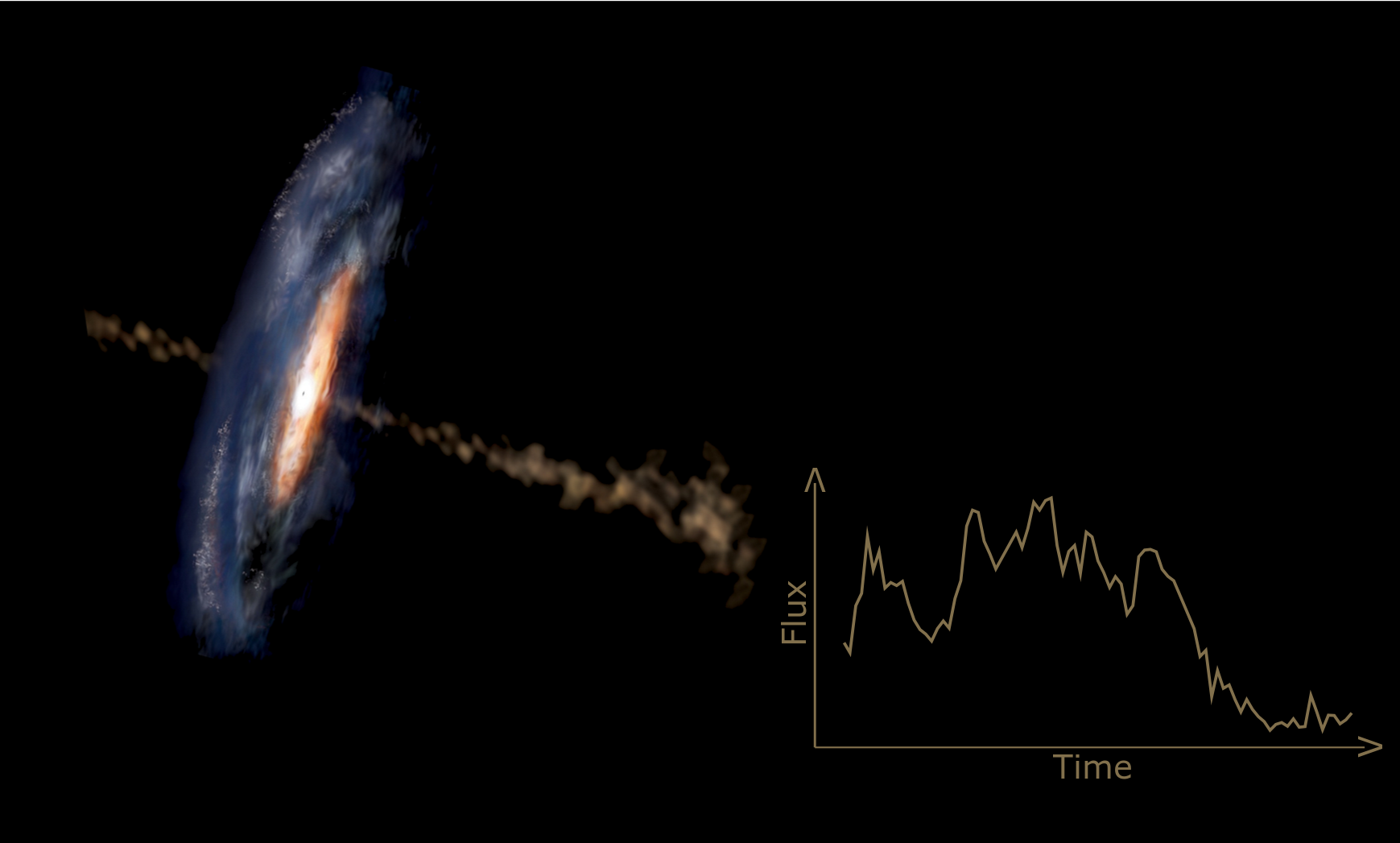
Up to now, the H.E.S.S. collaboration has published the detection of very-high-energy gamma-ray radiation from a total of 32 active galaxies (Fig. 2). Many of them were discovered to be very-high-energy gamma-ray emitters for the first time thanks to the H.E.S.S. AGN ToO program. Given the limited field-of-view of its telescopes, H.E.S.S. cannot continuously monitor the sky in very-high-energy gamma rays. Thus the H.E.S.S. ToO program relies on information about the activity of AGN from other telescopes in other wavelengths.
At lower gamma-ray energies the Fermi-LAT telescope [2] monitors the entire sky once every three hours. Whenever increased activity from any AGN observable with H.E.S.S. is detected, we trigger ToO observations of the object with H.E.S.S. and other telescopes across the electromagnetic spectrum, see Fig. 2. Besides these alerts from Fermi-LAT about gamma-ray activity of AGN, we may also receive alerts from other instruments in the very-high-energy gamma-ray band, e.g., the MAGIC and FACT telescopes on the Canary Island of La Palma (Spain), the VERITAS telescopes in Arizona (USA) and the HAWC observatory in Mexico.
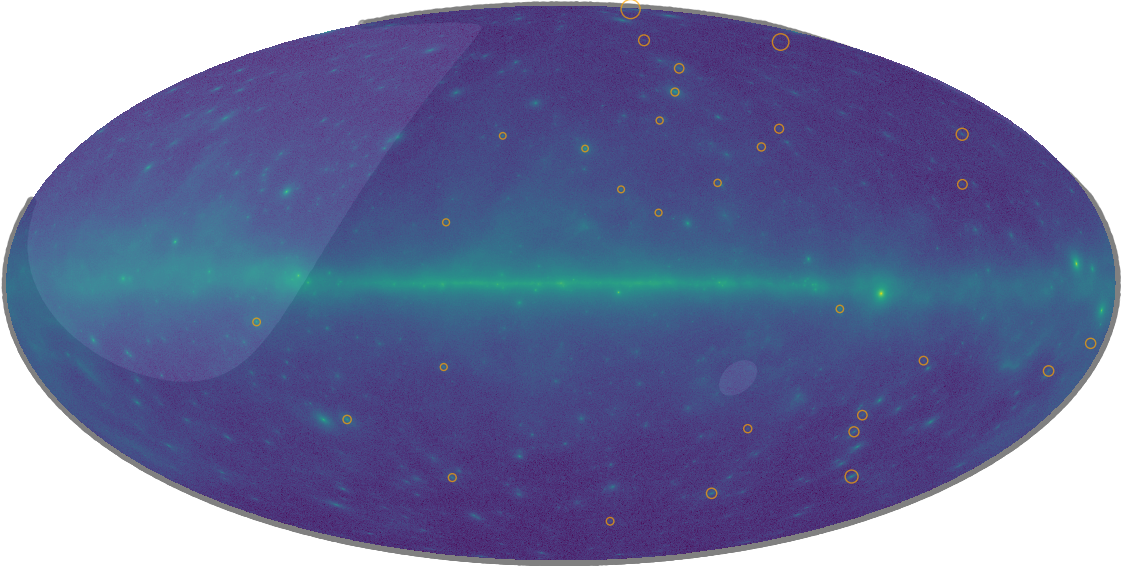
Brightness changes in AGN jets are usually not limited to just one single energy band, but instead often occur over the entire electromagnetic spectrum around the same time. Thus, we also react to alerts from optical (e.g., ATOM, Fig. 3, the optical support telescope at the H.E.S.S. site, H.E.S.S. SOM May 2020) and X-ray telescopes, such as the Neil Gehrels Swift Observatory [3].
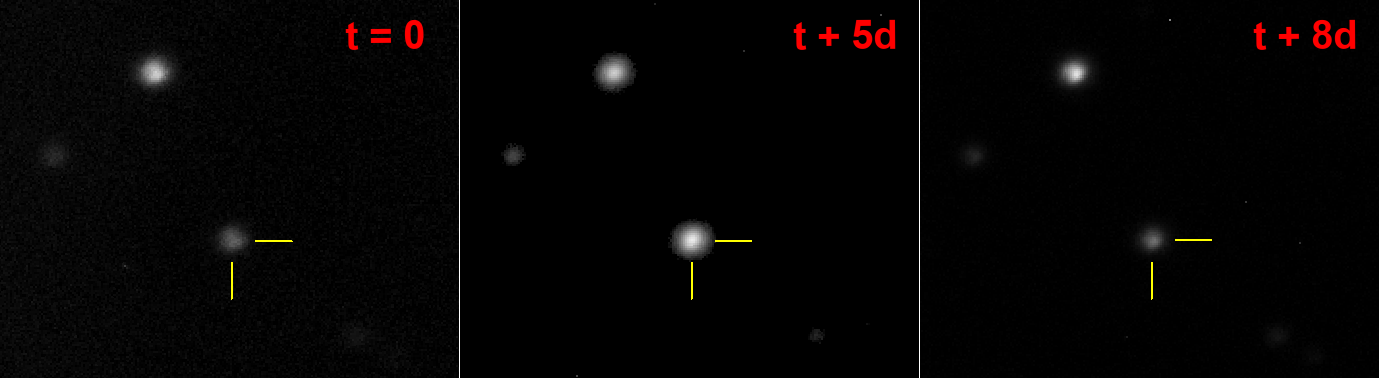
Dedicated software tools for the real-time detection of multiwavelength flaring activity of AGN and the rapid dissemination of such information to the world-wide network of observatories are essential for the success of the H.E.S.S. ToO program. These include FLaapLUC, an automated pipeline for the real-time analysis of Fermi-LAT data [4], Astro-COLIBRI [5], and the GCN [6] and AMON networks [7] for the rapid distribution of flare alerts.
The AGN ToO program allows us to discover very-high-energy emission from as-yet unknown gamma-ray AGN, as well as to deeply investigate elementary physics processes in AGN. A good sampling of observations around an outburst enables modelling of the acceleration of particles and the generation of high-energy radiation in the relativistic outflows of the AGN and regions close to the supermassive black hole. As the speed of light is finite, the duration of a brightening event contains information about the maximum size of the region where the gamma rays are produced. Such information can also be used to constrain the distance of that region from the central black hole.
While brightness variations usually happen across the entire electromagnetic spectrum, one can often observe time lags between variations in certain energy domains, and occasionally even "orphan flares" with the brightness varying only in one energy band but not in others. Such findings allow us to gain insights into the mechanisms with which particles are being accelerated to extreme energies and the processes that lead to the production of gamma rays.
Exotic topics beyond our well-understood standard models of physics can also be studied with such observations. For example, some models of quantum gravity (e.g., [8], [9]) predict that the speed of light is slightly different at different gamma-ray energies. Measurements of gamma-ray variability in different energy bands can test such predictions and thereby set limits on the relevant quantum-gravity mass scale (see [10], [11]).
One particular asset of the ToO program is that it gives us a chance to detect objects that would normally be too faint to be detectable in a quiescent state. This especially applies to objects which are far away enough that the photons we detect from them were produced during the "middle age" of the Universe, i.e., the time when the Universe was just about half its current age. The photons of such distant objects interact with light from stars and dust in galaxies throughout the Universe - the so-called extragalactic background light - on the way to Earth. These interactions produce electron-positron pairs absorbing the gamma rays and thus reducing the observed flux. The higher the energy and the further away the source of the photons, the stronger this effect is. With the very-high-energy gamma-ray detection of AGN at redshifts up to about 1, we can thus investigate this background light indirectly and explore how the Universe evolved over time.
Of course, these results would be impossible to obtain without a comprehensive picture of the event across a wide energy range and over a long time. The H.E.S.S. AGN ToO programme therefore routinely requests observations from other observatories like Swift, and the Southern African Large Telescope (SALT).
References:
[1] M. Urry & P. Padovani, 1995, Unified Schemes for Radio-Loud Active Galactic Nuclei, PASP, 107, 803
[2] https://fermi.gsfc.nasa.gov
[3] https://swift.gsfc.nasa.gov
[4] Lenain, J.-P., 2018, FLaapLUC: A pipeline for the generation of prompt alerts on transient Fermi-LAT gamma-ray sources, Astronomy and Computing, 22, 9–15
[5] Schüssler, F., et al., 2021, Astro-COLIBRI: a new platform for real-time multi-messenger astrophysics, PoS (ICRC2021) 935
[6] https://gcn.gsfc.nasa.gov/
[8] Mattingly, D., 2005, Modern tests of Lorentz invariance, Living Reviews in Relativity, 8, 5
[9] Liberati, S., 2013, Tests of Lorentz invariance: a 2013 update, Classical and Quantum Gravity, 30, 133001
[10] Aharonian, F., et al., 2008, Limits on an Energy Dependence of the Speed of Light from a Flare of the Active Galaxy PKS 2155-304, Phys. Rev. Lett., 101, 170402
[11] Abramowski, A., et al., 2015, The 2012 Flare of PG 1553+113 Seen with H.E.S.S. and Fermi-LAT, ApJ, 802, 65A