The biggest cluster blows an energetic bubble
August 2022
For more than 100 years, it is known that cosmic rays (charged particles) are accelerated to extremely high energies somewhere in the Milky Way. And yet, we have still not uncovered their origin unambiguously. Whilst young and powerful supernova remnants (SNRs) have long been postulated to be responsible, young massive star clusters are becoming increasingly popular as potential major contributors to the flux of Galactic cosmic rays (see, for example, [1]).
Westerlund 1, which was discovered by Bengt Westerlund in 1961 [2], is the most massive known young stellar cluster in our Galaxy (for a review about massive stellar clusters, see [3]). This makes it a prime target for testing the stellar cluster hypothesis. It contains a large number of very massive stars, among them a significant fraction of all known Yellow Hypergiants and Wolf-Rayet stars. It is most likely located only ~4 kpc away from Earth, its total mass is about 105 solar masses (half of which is located within a radius of ~1 pc), and its age is estimated to lie between 3 and 5 million years.
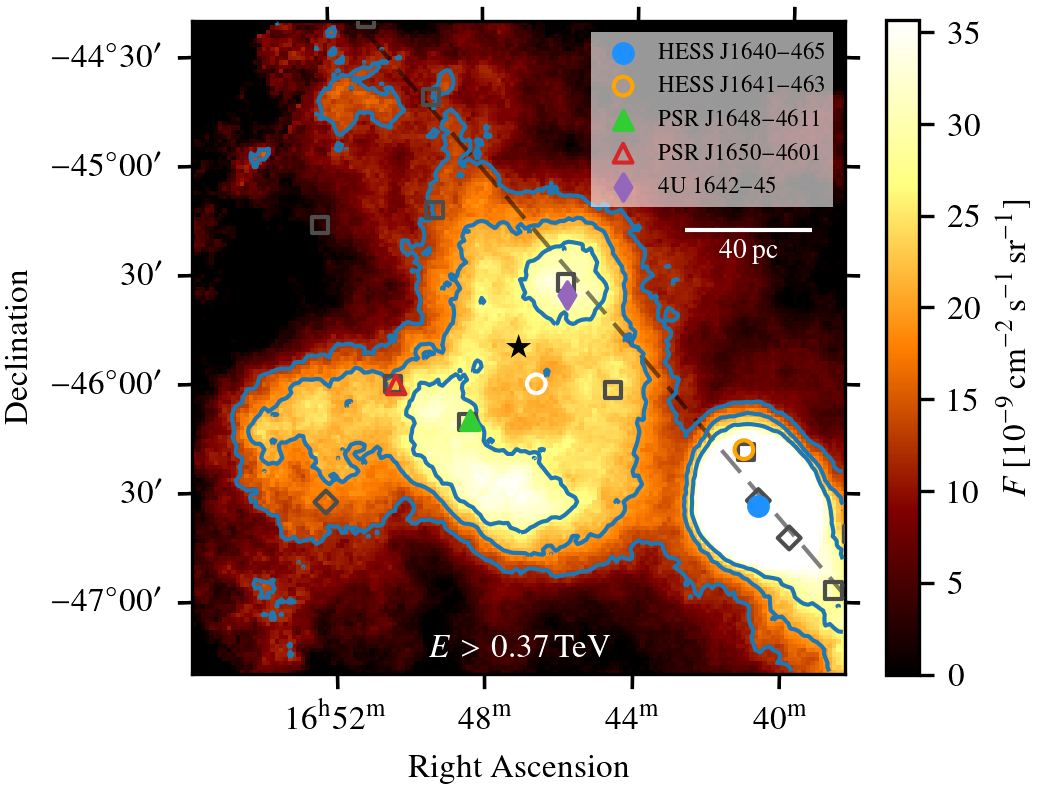
In 2012, H.E.S.S. reported the detection of HESS J1646–458 [4]. This is a largely extended gamma-ray source which is spatially coincident with Westerlund 1, but that extends far beyond the stellar cluster itself (see also the previous SOM June 2009). However the emission could not definitively be connected with Westerlund 1 at the time, possibly due to the limited amount of data. Since 2012, the H.E.S.S. exposure time on this source has almost quintupled. This now enabled us to perform a much more detailed analysis of the gamma-ray emission which was accepted for publication end of July 2022 [5].
In Fig. 1 we show a flux map of the gamma-ray emission of HESS J1646–458. The source extends over a region almost 2° across, which corresponds to about 140 pc at the assumed distance to Westerlund 1. The morphology of the source is quite complex. Remarkably, the emission is not peaked at the position of Westerlund 1, but rather exhibits a shell-like structure with several additional bright peaks. The centre of the shell structure is offset from the position of the cluster by ~11'.
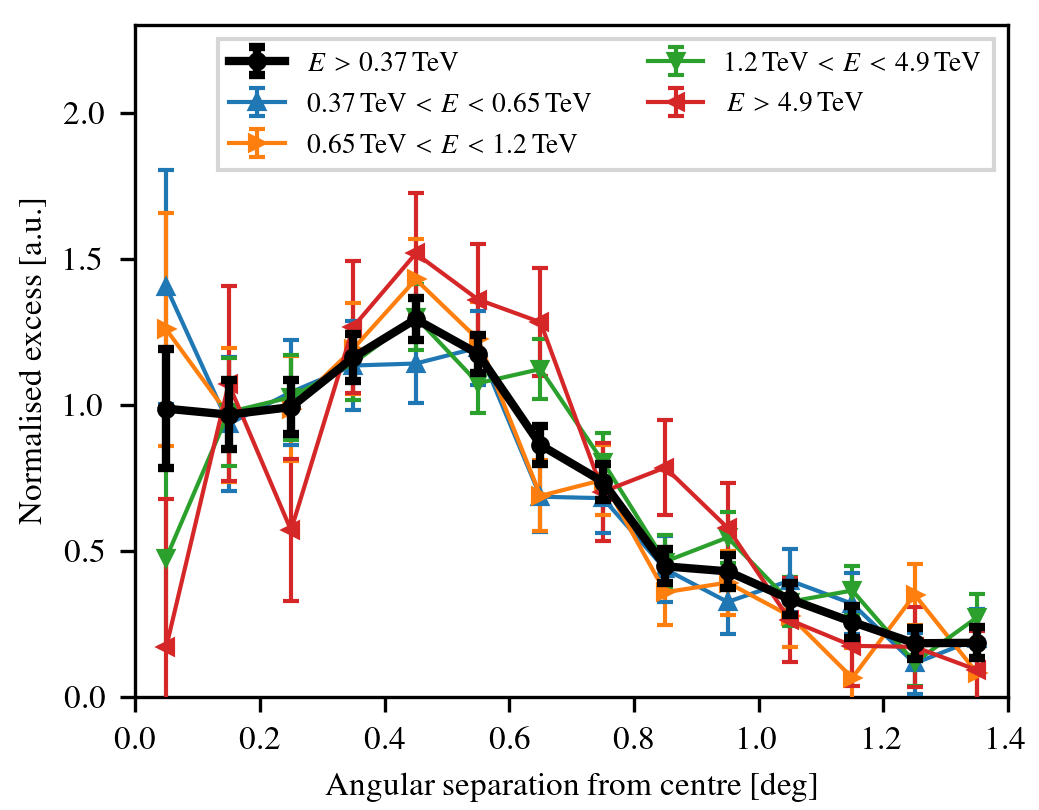
The shell-like structure is also evident in the peak visible in the radial excess profile at 0.45 deg angular separation from the centre that is shown in Fig. 2. This structure does not change with energy; the profiles derived for separate energy bands are all compatible with each other. This observation has important implications for the possible origin of the emission. In particular, it essentially rules out any model in which cosmic-ray electrons are accelerated within a small volume inside the source, since one would then expect to observe the size of the source to shrink at higher energies. This also rules out the pulsars PSR J1648–4611 and PSR J1650–4601 , indicated on the map in Fig. 1 as dominant sources of the gamma-ray emission. This leaves only Westerlund 1 as a realistic association of HESS J1646–458.
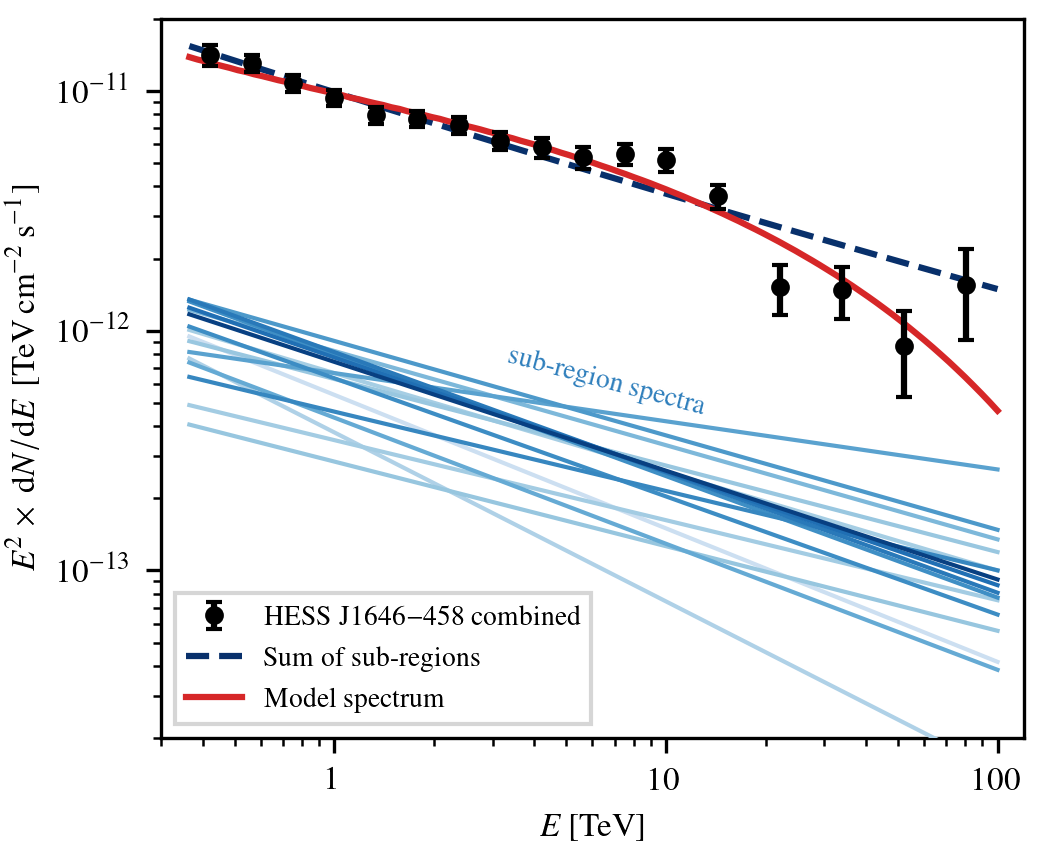
Finally, the combined energy spectrum of HESS J1646–458 is displayed in Fig. 3. It extends to nearly 100 TeV, which unveils that Westerlund 1 is acting as a powerful cosmic-ray accelerator. The spectrum can be fitted well with models that explain the gamma-ray emission as originating from both, cosmic-ray protons or electrons (both models have nearly indistinguishable spectra when fit to the data.)
Cosmic rays could be accelerated by Westerlund 1 at several different sites. The acceleration could happen at shocks that form when the intense winds of the massive stars in the cluster collide. However, the fact that the gamma-ray emission is not peaked at the cluster position disfavours this scenario. It seems more likely that the acceleration is linked to the collective cluster wind, which forms as a superposition of the winds of all the stars in the cluster and blows a superbubble into the interstellar medium. One possible acceleration site that has recently been put forward is the termination shock of the collective wind, which forms where the pressure exerted by the wind equals that of the surrounding medium (e.g., [6]). While we cannot firmly associate the gamma-ray emission with the termination shock, it is intriguing that the expected distance of the termination shock from the cluster matches the radius of the shell-like structure observed in gamma rays with H.E.S.S., which supports this model. This suggests for the first time that the collective cluster wind of a massive star cluster is directly linked to high-energy gamma-ray emission, and can thus be identified as a cosmic-ray acceleration site.
References:
[1] F. Aharonian, R. Yang, E. de Oña Wilhelmi, Nature Astronomy
[2] B. Westerlund, Publications of the Astronomical Society of the Pacific
[3] S. F. Portegies Zwart et al., Annual Review of Astronomy and Astrophysics
[4] A. Abramowski et al. (H.E.S.S. Collaboration), Astronomy & Astrophysics
[5] F. Aharonian et al. (H.E.S.S. Collaboration), accepted in Astronomy & Astrophysics (2022). arXiv:2207.10921
[6] G. Morlino, P. Blasi, E. Peretti, P. Cristofari, Monthly Notices of the Royal Astronomical Society