On HESS Follow-up observations of the Brightest Gamma-ray Burst Of All Time a.k.a. the BOAT
April 2023
It was a mind-boggling explosion that happened 3 billions years ago in a distant galaxy. The extremely energetic flash emitted with the explosion traveled at the speed of light and hit Earth in October 2022, and was recorded as the brightest gamma-ray burst (GRB) that has ever been detected.
GRBs are observed as bright X-ray and gamma-ray flashes from distant sources outside of our Galaxy. They come from the deaths of rapidly rotating massive stars and the mergers of compact objects such as neutron stars. For the first kind of GRB, the core of the star collapses and a fraction of the released gravitational energy is fed into a violent blast wave ploughing through the remnants of the star at nearly the speed of light. The resulting electromagnetic emission can be roughly divided into two phases: the prompt phase, the initial bright flashes that typically last up to a few tens of seconds, and the slowly fading afterglow phase that can be detectable over a large part of the electromagnetic spectrum for days or months. During the afterglow, the blast wave produces relativistic shocks that propagate into the surrounding material and accelerate charged particles such as electrons. These accelerated electrons then interact with the magnetic field in the material, emitting X-ray radiation in the form of synchrotron radiation.
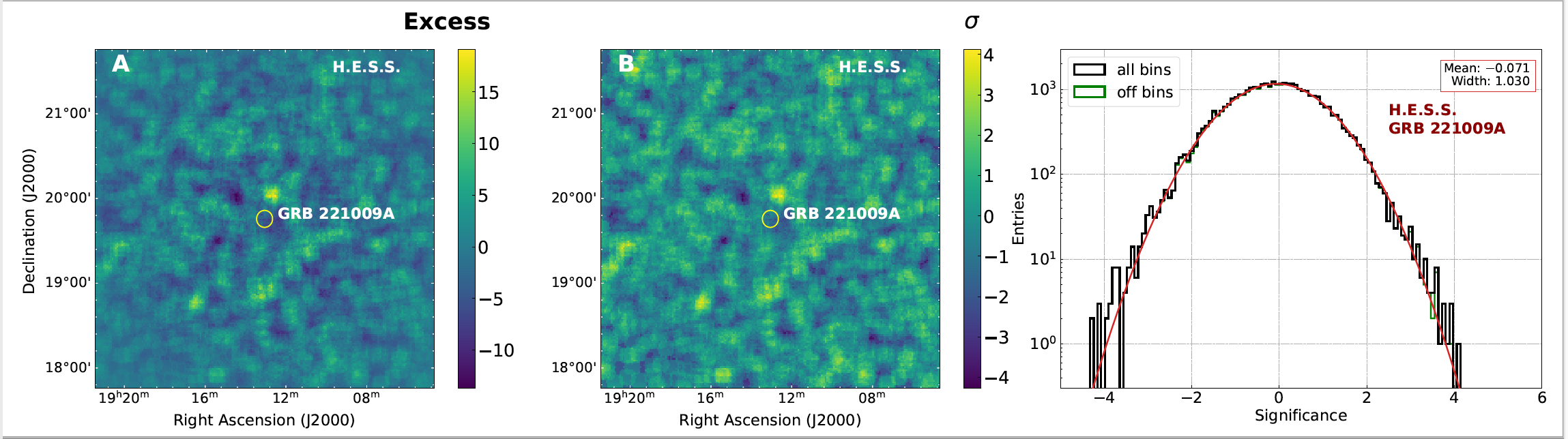
The physics of GRB afterglows is a prime opportunity for the study of relativistic shocks. The synchrotron emission process, which produces the X-ray emission, has been studied extensively and is well understood; however, the details of emission at higher energies, such as the very-high energy (VHE) gamma-ray (> 100 GeV) domain, is still under debate. In the last few years, a number of GRBs have been detected in VHE gamma rays. This VHE component is usually associated with the inverse Compton scattering of either ambient or synchrotron photons, the latter being known as the synchrotron self-Compton (SSC) scenario. However, a mismatch between observations and the usually employed single-zone (i.e., uniform magnetic field) SSC description was noted in GRB 190829A (see [1] and the June 2021 SOM). Further observations, especially in the VHE gamma-ray domain, are therefore necessary to shed light onto this open question.
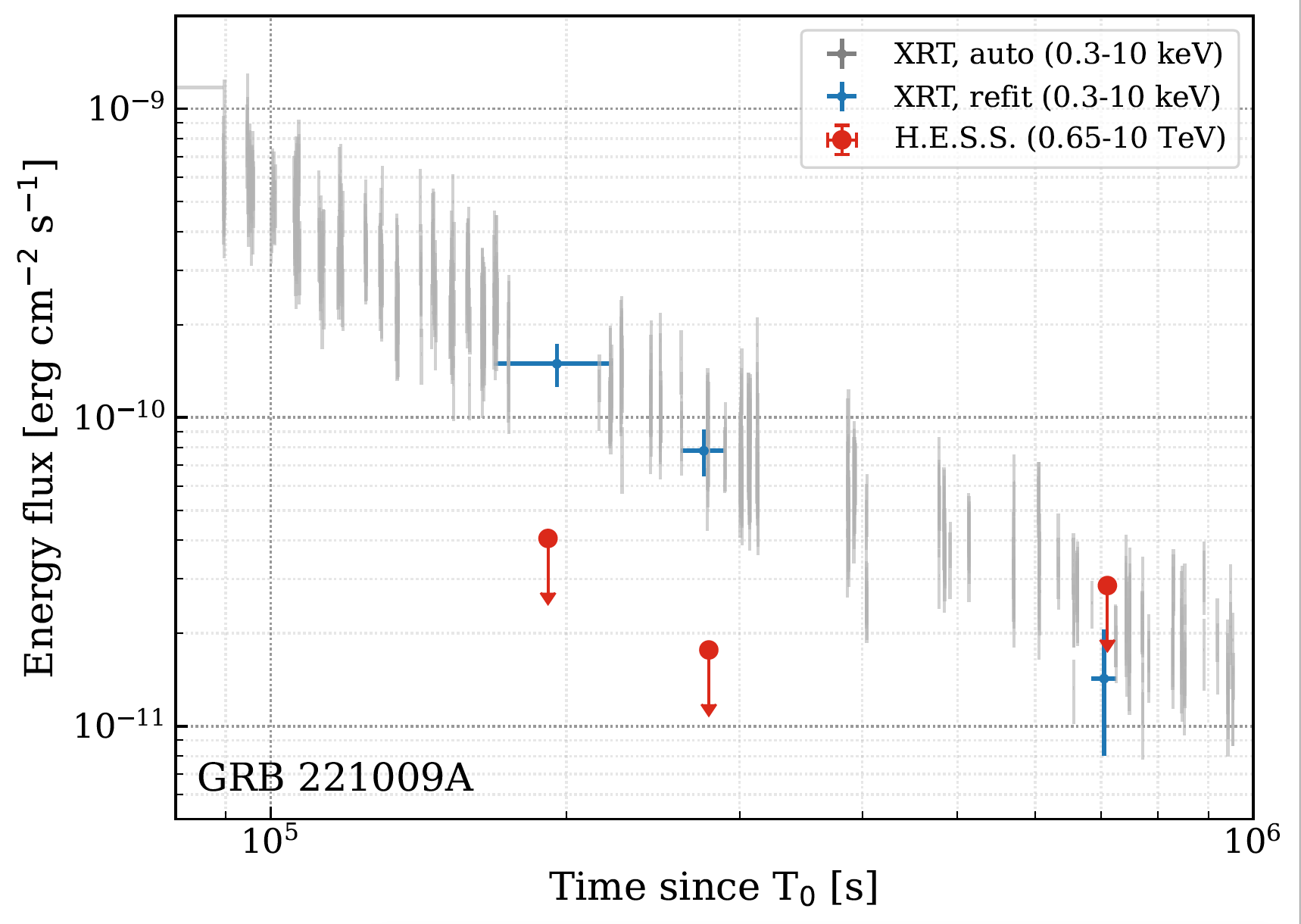
On October 9th 2022, the Fermi Gamma-ray Burst Monitor and later the Neil Gehrels Swift Burst Alert Telescope detected and localized GRB 221009A. H.E.S.S. was not able to observe the burst immediately as, due to the full moon at the time, the night sky background was too high for the operation of our highly sensitive instruments. However, observations become possible two days later, on October 11, and continued over more than a week after the burst was first detected. Unfortunately, the campaign was heavily influenced by rather poor atmospheric conditions including cloudy skies and a high aerosol content. Careful analyses of the data acquired by H.E.S.S did not yield any significant detection of VHE gamma rays at the GRB position in the total dataset nor for individual nights (cf. Fig. 1). Nevertheless, we were able to derive stringent upper limits on the VHE gamma-ray flux. These can be put into the context of the X-ray afterglow (cf. Fig. 2) and are useful to constrain the various possible theoretical emission scenarios. An example for this is given in Fig. 3.
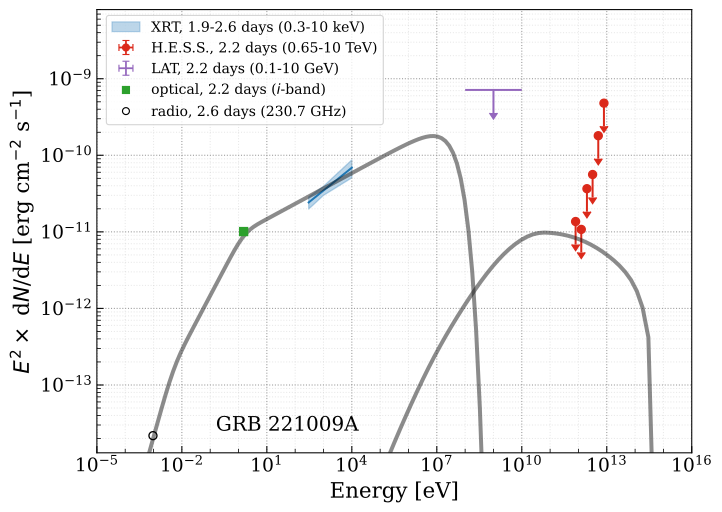
GRB 221009A had many exceptional features in addition to its exceptional brightness. In particular, the long lived X-ray afterglow spectrum remained consistently hard for several nights; i.e., in the X-ray regime, a larger fraction of the total energy was emitted in higher-energy photons compared to lower-energy photons than is often seen for GRBs at these times. Such hard spectra are frequently observed at low frequencies such as optical or radio, due to the accumulation of energetic electrons that have not yet cooled appreciably. It is however rare for X-ray emission to be both bright and ineffectively cooled at such late times. A possibility is that the electrons themselves are continuously accelerated with a remarkably hard spectrum. Why this should be the case is a mystery. A firm detection by H.E.S.S. in such cases would greatly advance our understanding of these rare events, but the upper limits equally play an important role in disentangling the various theoretical models. An important question that remains to be answered concerns why some afterglows are gamma-ray bright with comparable X-ray and gamma-ray fluxes (e.g. GRB 190829A), while for others, like GRB 221009A, the VHE emissions seems evidently much weaker. VHE gamma-ray astronomy has a major role to play in this story.
References:
[1] Abdalla et al. (H.E.S.S. Collaboration). 2021, Revealing x-ray and gamma ray temporal and spectral similarities in the GRB 190829A afterglow,Science, 372, 1081
[2] Evans, P. A., Willingale, R., Osborne, J. P., et al. 2010, The Swift Burst Analyser I. BAT and XRT spectral and flux evolution of gamma ray bursts,A&A, 519, A102
[3] Rhodes, L., Huang, K., & Cendes, Y. 2022, GRB 221009A/Swift J1913.1+1946: SMA observations, GRB Coordinates Network, 32707, 1
[4] Fulton, M. D., Smartt, S. J., Rhodes, L., et al. 2023, The optical light curve of GRB 221009A: the afterglow and detection of the emerging supernova SN 2022xiw
[5] F. Aharonian et al. (H.E.S.S. Collaboration), H.E.S.S. follow-up observations of GRB 221009A, accepted for publication in APJ Letters (2023)