Dark matter in Irregular galaxy "WLM"
January 2021
For many decades now scientists have been searching for a new form of matter, which is believed to be ubiquitous in the Universe from cosmological to galactic scales. This invisible form of matter is called dark matter and represents 85% of the total matter of the Universe. Several hints suggest its existence. The first evidence was found by Fritz Zwicky in 1933 who showed that the total mass of the Coma galaxy cluster was about 500 times greater than the combined mass of all individual galaxies it contained [1],[2]. The presence of dark matter in the Universe could also bring a solution to explain the formation of large scale structures, the motion of stars in galaxies, and the bending of light propagating near massive objects.
The nature of dark matter still remains a mystery to this day and its discovery represents one of the biggest challenges of modern fundamental physics. Although we do not know much about its nature, some of its properties can be inferred from observations. Dark Matter is not made out of the same elementary particles that we have identified in laboratories on Earth. This form of matter interacts mostly gravitationally, and is thus difficult to detect directly. In addition, dark matter is thought to be massive to make up for all the mass, electrically neutral, with non-relativistic velocities, and collisionless with ordinary matter. The best candidates gathering all of these properties are called the WIMPs for Weakly Interacting Massive Particles. When two WIMP particles interact, they annihilate and produce indirect evidence for dark matter by creating ordinary particles such as gamma rays, which can be detected by our telescopes [3].
Dark matter is expected to be present in large quantities in dense regions such as dwarf galaxies or the Galactic center. Extensive searches have been conducted with the H.E.S.S. telescopes as well as instruments sensitive to gamma rays of lower energies (e.g. by using data obtained with the Fermi satellite). Previous searches have been described in earlier reports of the sources of the month (H.E.S.S. SOM 8/2019, H.E.S.S. SOM 2/2018, H.E.S.S. SOM 11/2016, or H.E.S.S. SOM 3/2016) and mostly covered the center of our Milky Way galaxy or nearby dwarf spheroidal galaxies.
The dynamics of galaxies reflects their formation history and cosmic evolution. Since dynamics is also used to indef the amount of Dark Matter, it is important to search for dark matter in different kind of galaxies, and the HESS collaboration has extended its earlier studies to so-called dwarf irregular galaxies, and, in particular, to the nearby, prototypical object, the WLM galaxy.
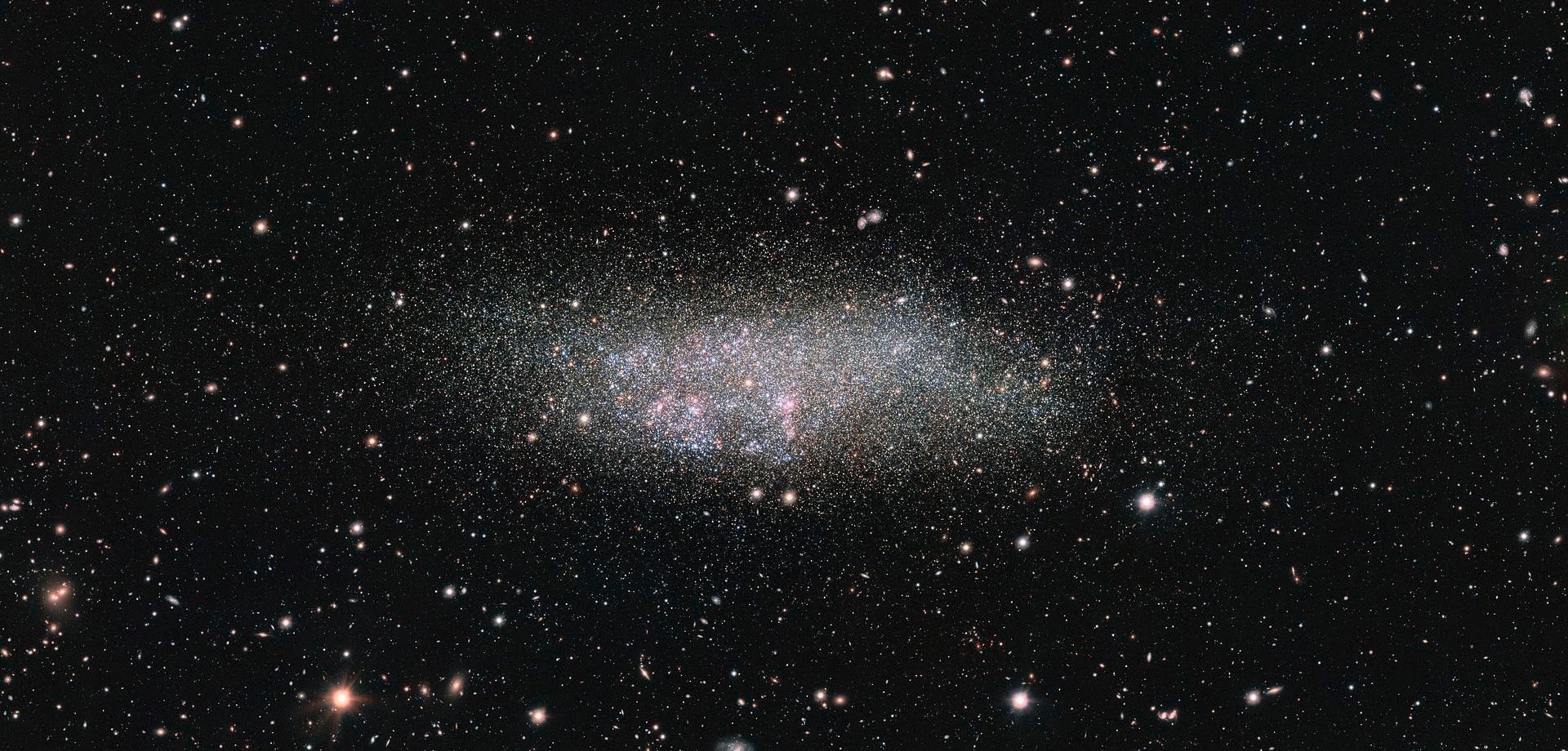
WLM is classified as a so-called 'dwarf irregular galaxy'. It was discovered by Max Wolf in 1909 using the telescopes of his observatory on the Königstuhl in Heidelberg [4] and identified as a dwarf galaxy by Knut Lundmark and Philibert Jacques Melotte seven years later. This dwarf galaxy is of oblate spheroidal shape [5], viewed under a high inclination of about 74 degrees [6]. It is located in the outer parts of the Local Group at a distance of 990 kpc (3.2 millions light years) and has an optical size of about 2.5 kpc (~8,100 light years) at its greatest extent.
WLM is believed to have developed away from other astrophysical systems since it does not present any sign of deformation [7]. It contains a smooth gas distribution with well-measured photometry and stellar kinematics [6],[8] used to constrain its internal dynamics and its dark matter distribution. Since this dwarf galaxy still actively forms stars at its center, several cycles of star formation and supernova explosions have occurred during its lifetime. These astrophysical processes have a consequence on the dark matter distribution of the dwarf, potentially lowering the dark matter density at its core as the matter gets ejected to the outer part.
WLM was observed by the five telescopes of the H.E.S.S. array between October and December 2018 in an attempt of capturing a dark matter annihilation signal, with a total of about 18 hours of high quality data. The data does not show any sign of such annihilation signal from dark matter as shown on Figure 2
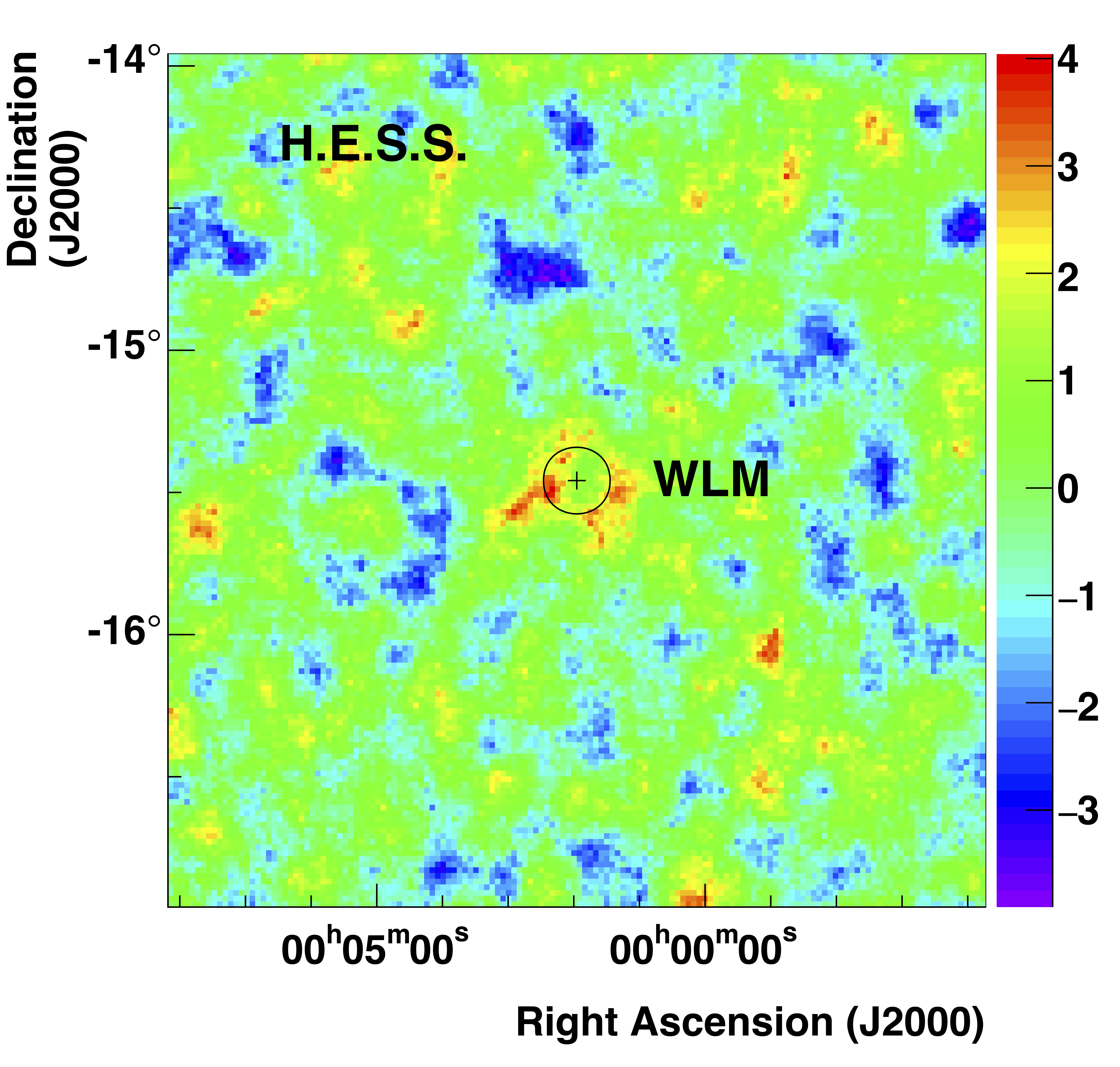
Although there is no dark matter detection, constraints on its annihilation cross section can be derived for each mass assumption. The derivation of such constraints relies on the computation of the amount of dark matter particle annihilations. This quantity is robustly derived using the well-measured rotation curve of WLM with smaller uncertainties than previous work.
Figure 3 presents an example of upper limits in the case of an annihilation of the WIMP particles into the τ + τ - and W + W - channels. All values above the limits are considered forbidden, otherwise we would have detected dark matter, while all values below the limits are still allowed, as our telescope sensitivity does not allow us to explore more constraining values. The limits are expressed in term of the annihilation cross section <σv>, which is an indicator of the annihilation rate of the dark matter particles.
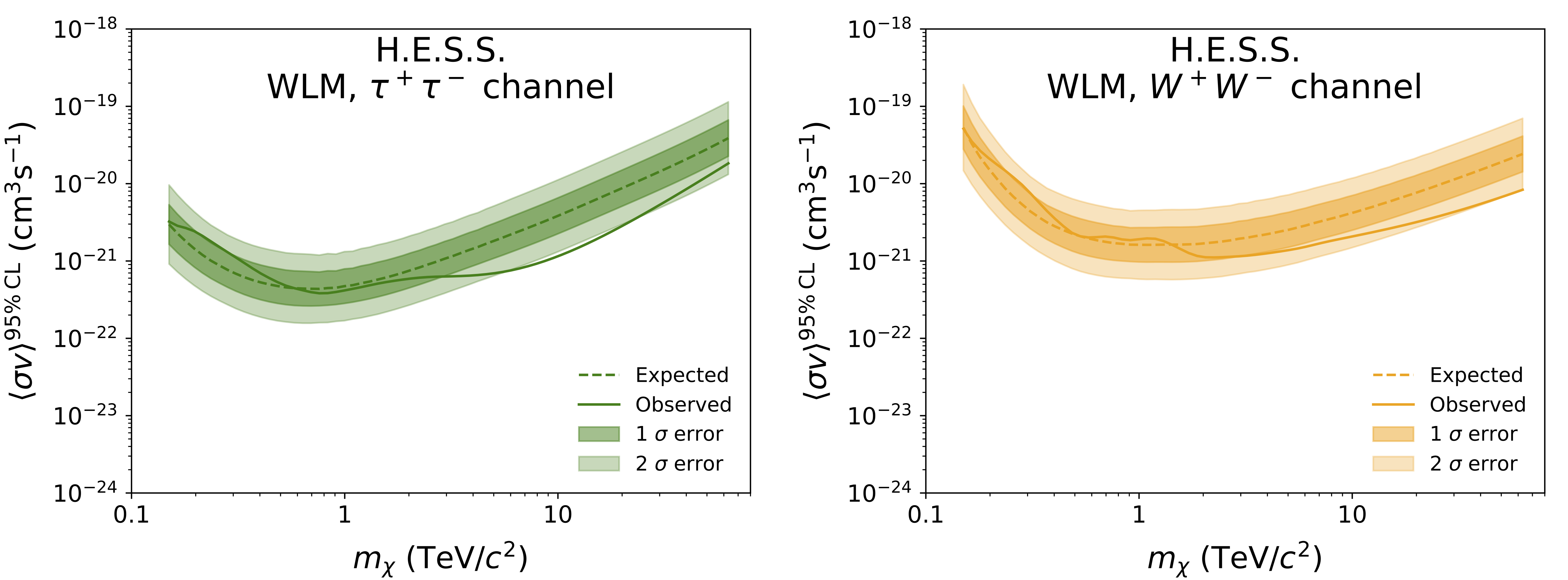
Using the data collected in the direction of WLM, the H.E.S.S. collaboration improves the constraints on the dark matter annihilation cross section by a factor of at least 10 to more than 200 compared to those of the HAWC experiment Figure 4.
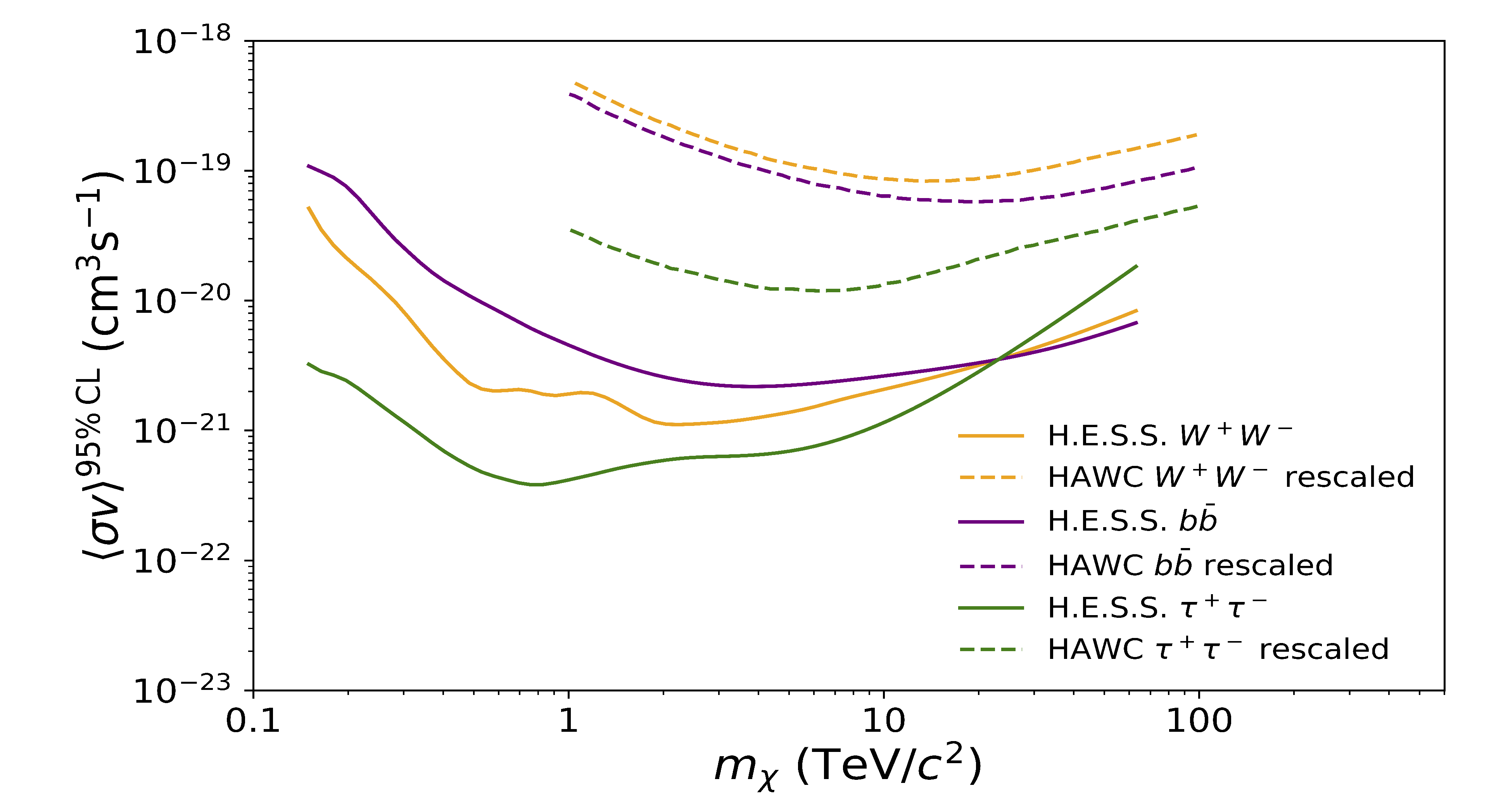
The WLM object represents one of the most promising dwarf irregular galaxies for dark matter search. Its rotation curve is well-measured leading to a robust reconstruction of its dark matter density profile. As no significant excess has been detected in the direction of WLM, constraints on the annihilation cross section of dark matter have been derived for several annihilation channels. The constraints obtained by the H.E.S.S. collaboration improve compared to those obtained by the HAWC experiment. Dwarf irregular galaxies are complementary targets to dwarf spheroidal galaxies and provide an alternative dark matter target for the next generation of Cherenkov telescopes.
References:
[1] Zwicky, Fritz, 1933. Die Rotverschiebung von extragalaktischen Nebeln. Helv. Phys. Acta, Vol. 6, 110–127. DOI = 10.1007/s10714-008-0707-4.
[2] Zwicky, Fritz, 1937. On the Masses of Nebulae and of Clusters of Nebulae. Astrophys. J. Vol. 86, 217–246. DOI = 10.1086/143864.
[3] Feng, Jonathan L., 2010. Dark Matter Candidates from Particle Physics and Methods of Detection. Ann. Rev. Astron. Astrophys. Vol. 48, 495–545. DOI = 10.1146/annurev-astro-082708-101659.
[4] Wolf, Max, 1909. Über einen größeren Nebelfleck im Cetus. Astronomische Nachrichten, Vol. 183, No. 4380, col. 187/188.
[5] Leaman, Ryan, et al., 2012. The resolved structure and dynamics of an isolated dwarf galaxy: a VLT and Keck spectroscopic survey of WLM. Vol. 750, No. 1. DOI = 10.1088/0004-637x/750/1/33.
[6] Read, Justin, et al., 2016. Understanding the shape and diversity of dwarf galaxy rotation curves in ΛCDM. Mon. Not. Roy. Astron. Soc. Vol. 462, No. 4, 3628-3645. DOI = 10.1093/mnras/stw1876.
[7] Rejkuba, Marina, et al., 2000. Deep Hubble Space Telescope STIS Color-Magnitude Diagrams of the Dwarf Irregular Galaxy WLM: Detection of the Horizontal Branch. Astron. J. Vol. 120. No. 2, 801–809. DOI = 10.1086/301501
[8] Read, Justin, et al., 2018. Dark matter heats up in dwarf galaxies. Mon. Not. Roy. Astron. Soc. Vol. 484, No. 1, 1401–1420. DOI = 10.1093/mnras/sty3404
[9] Cadena, Sergio, et al., 2018. Searching for TeV DM evidence from Dwarf Irregular
Galaxies with the HAWC Observatory. ICRC 2017. Pages 897. DOI = 10.22323/1.301.0897.