Dark Matter Search In The Inner Galactic Halo: An Update With Ten Years Of Data
March 2016
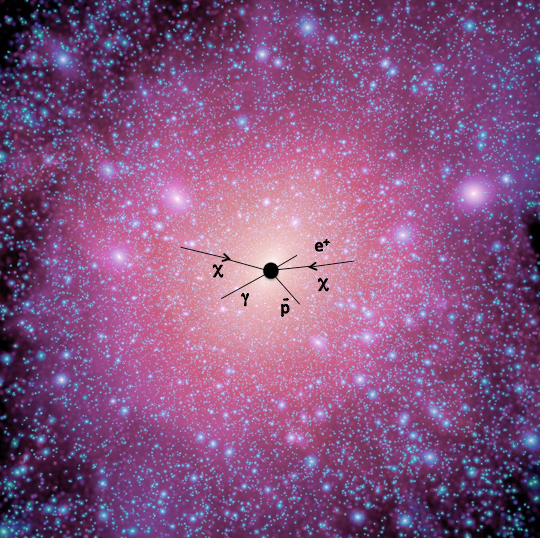
It is a widely accepted idea that dark matter, which contributes about 27% to today’s total energy content of the universe, consists of elementary particles. So far, dark matter agglomerations have been detected only very indirectly due to the impact of their mass on the dynamics of the surroundings. One example is the fact that the observed velocity of stars in some galaxies is too large to keep them bound to the galaxy by gravity, if only the mass of visible matter is accounted for.
Since dark matter particles have not been directly detected so far, they must be electrically neutral, stable (or have a lifetime exceeding the age of the universe) and non-baryonic. Furthermore, observations of the matter distribution in the universe and simulations of their structure formation tell us that a large portion of the dark matter must be in the form of massive, i.e. non-relativistic, particles, termed cold dark matter. Among the well-motivated elementary particle candidates for cold dark matter are so-called weakly interacting massive particles (WIMPs); these are (quasi-)stable particles which are supposed to interact only via the weak interaction, with masses and coupling strengths at the electroweak scale. If produced in a standard thermal history of the Universe, such WIMPs would have a relic density matching well today's observed dark-matter density. Their inferred velocity-weighted annihilation cross section – a measure for the probability that two dark-matter particles close to each other would annihilate – is about 3x10-26 cm3s-1. This cross section is often referred to as the "natural scale" to probe for their existence in indirect dark-matter searches. In these searches, one looks for the presence of stable standard model particles produced from the annihilation process, e.g. high-energy gamma rays or neutrinos.
The number of gamma rays observable from dark-matter annihilations does not only depend on the annihilation cross section, but also on the square of the number density of the dark-matter particles. Therefore, regions in the sky that have a putatively large dark matter density are most promising targets for dark matter searches. As suggested by simulations, this is particularly true for the centers of galaxies where the largest dark matter density is expected (Fig. 1). It is for this reason that the central region of our own galaxy (the Galactic Centre, GC) is a prime target for dark matter searches. Furthermore, since the gamma-ray intensity decreases with increasing target distance, the GC has the particular advantage of being located close to Earth (at a distance of 8.5 kpc).
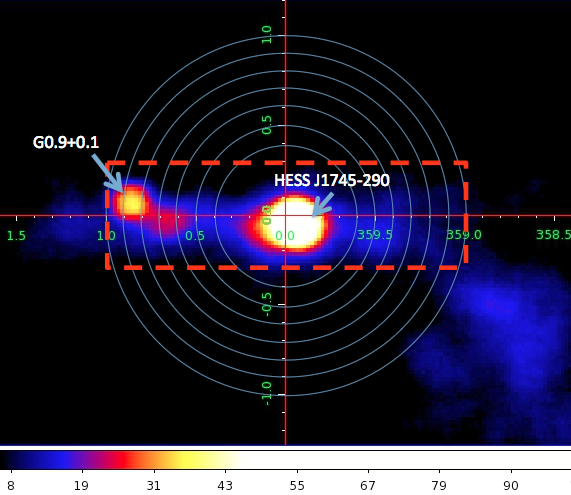
The inner 300 parsecs region of the Milky Way has been observed by the H.E.S.S. telescopes since 2004, making it one of the regions with the largest exposure to search for very-high-energy (VHE, E > 100 GeV) emission. Several VHE gamma-ray sources have been discovered in the GC region, such as HESS J1745-290 spatially coincident with the supermassive black hole Sagittarius A*, the pulsar wind nebula inside the supernova remnant G0.9+0.1, and a band of diffuse emission along the Galactic plane. Therefore, while dark matter signals from the GC are expected to be larger than those from nearby galaxies by orders of magnitude, searches for these signals in the GC region face a strong standard astrophysical background (as opposed to other targets such as dwarf galaxies which are essentially free of these backgrounds).
A 10-year monitoring during the years 2004-2014 of the GC with the H.E.S.S. phase-1 array results accumulated a total exposure time of 254 hours. This is more than twice the exposure used in a previous H.E.S.S. search for dark-matter annihilations in that region. Much like the previous search, the analysis presented here makes use of a circular search region with a radius of 1° centered on the Galactic Center (Fig. 2). However, contrary to the previous search, the sensitivity of this work is improved by taking full advantage of the fact that both the energy and spatial distribution of the expected gamma-ray emission due to dark matter annihilation in the search region - which for instance is expected to be strongly peaked towards the center - are much different from any expected background emission. To this end, the search region is subdivided into concentric annuli of of 0.1° width; for each of these, the expected gamma-ray emission due to dark matter annihilation is compared to the data for different assumptions on the annihilation cross section, the dark matter particle mass and the physics of the annihilation process. The region along the Galactic plane dominated by standard astrophysical emission (red rectangle in Fig. 2) is excluded from the analysis.
The data analysis does not show any significant gamma-ray excess above
the expected background in any of the annuli. Therefore, constraints
on the DM velocity weighted annihilation cross section
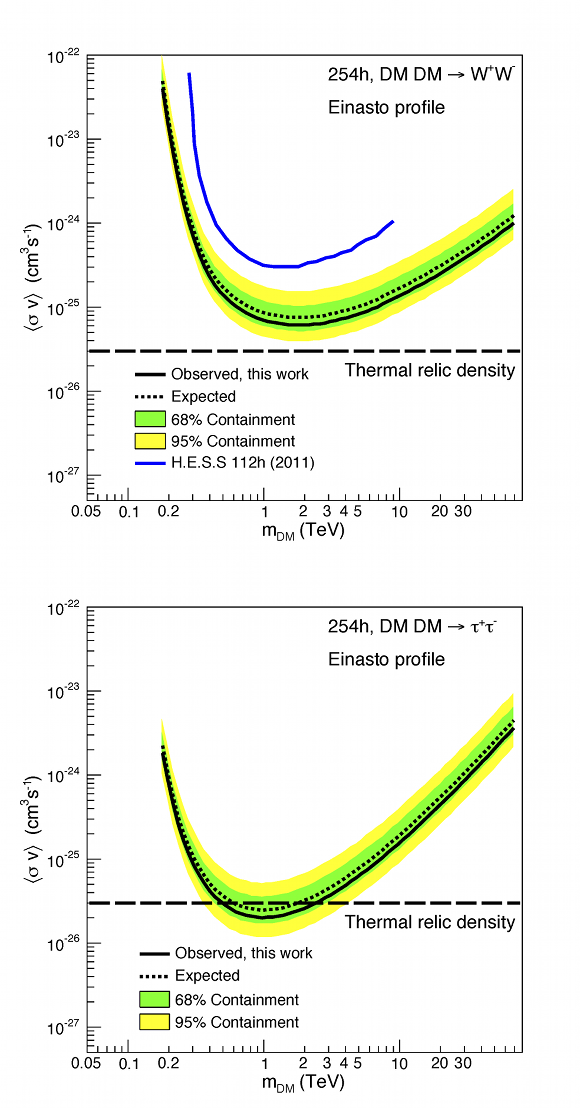
Reference: Abramowski et al. (H.E.S.S. Collaboration), "Search for dark matter annihilations towards the inner Galactic halo from 10 years of observations with H.E.S.S.", in preparation. Currently undergoing internal collaboration review prior to submission.