The Black Hole in the Galactic Centre
November 2020
On October 6th 2020 the Royal Swedish Academy of Sciences has decided to award the Nobel Prize in Physics 2020 with one half to Roger Penrose “for the discovery that black hole formation is a robust prediction of the general theory of relativity” and with one half jointly to Reinhard Genzel and Andrea Ghez “for the discovery of a supermassive compact object at the centre of our galaxy”. The H.E.S.S. collaboration congratulates our colleagues. The Nobel Prize in physics is an outstanding recognition of the scientific achievements of the Laureates which, in turn, have long been an important stimulus for scientific work in many fields of astrophysics – including, in particular, the science programme of the H.E.S.S. collaboration.
Quoting the announcement of the Swedish Academy of Sciences "January 1965, (...) Roger Penrose proved that black holes really can form and described them in detail; at their heart, black holes hide a singularity in which all the known laws of nature cease" [1]. In the wake of the seminal paper by Roger Penrose, the then newly-discovered Quasars, sites of the highest energy densities in the Universe, were speculated to be driven by very massive Black Holes, a speculation that is now generally accepted. Quasars are the most numerous sources of very high energy gamma-ray emission known and the fascinating processes in the vicinity of supermassive Black Holes that make them such efficient particle accelerators and emitters of non-thermal Gamma-ray radiation are a major motivation for the H.E.S.S. collaboration and other researchers investigating the high-energy universe.
To a large extent it was the work of the other two recipients of this years' Nobel Prize, that convinced the scientific community of the existence of a supermassive Black Hole in the center of our Milky Way galaxy. Again quoting the Committee's appraisal: "Reinhard Genzel and Andrea Ghez each lead a group of astronomers that, since the early 1990s, has focused on a region called Sagittarius A* at the centre of our galaxy. The orbits of the brightest stars closest to the middle of the Milky Way have been mapped with increasing precision. The measurements of these two groups agree, with both finding an extremely heavy, invisible object that pulls on the jumble of stars, causing them to rush around at dizzying speeds. Around four million solar masses are packed together in a region no larger than our solar system. (...) Their pioneering work has given us the most convincing evidence yet of a supermassive black hole at the centre of the Milky Way" [1].
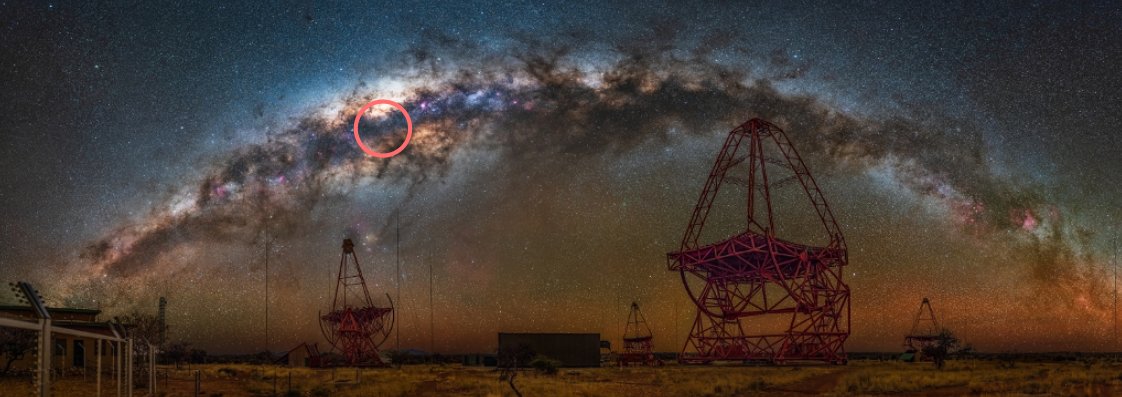
The centre of the Milky Way is strongly obscured by dense clouds of gas and dust, which prohibit direct observations in visible light and other parts of the electromagnetic spectrum. Radio waves, which penetrate dust rather easily, had revealed a compact radio source, identified as Sagittarius A* in the direction towards the Centre of the Milky Way. It remained an obvious target for studies throughout the electromagnetic spectrum, and had also been identified as a gamma-ray source with the Whipple 10m-telescope, a pioneering instrument among the previous generation of ground-based gamma-ray telescopes [2]. Straight after the start of H.E.S.S. operations, Sagittarius A* was observed. While immediately confirming a source of gamma-ray radiation in the direction of the Galactic Centre, classified as HESS J1745-290 [3] (see also H.E.S.S. SOM 12/2004), understanding the nature of the very high energy gamma-ray emission and its potential association with the Black Hole source Sagittarius A* remain challenging. The most recent studies acknowledged and honored with last month's Nobel Prize in Physics lead the most promising path yet for further progress.
The angular resolution of the H.E.S.S. telescope array is best among gamma-ray instruments but does not match the exquisite resolution obtained with Chandra, the VLTI or radio interferometers in the X-ray, near-infrared and radio bands, respectively. It is, unfortunately, insufficient to spatially associate the source HESS J1745-290 uniquely in the very dense environment of the Galactic Centre. This led to many competing explanations for the nature of the gamma-ray emission of HESS J1745-290, ranging from Pulsar Wind Nebulae to spikes of dark matter that radiate gamma-rays resulting from annihilating Dark Matter particles [4].
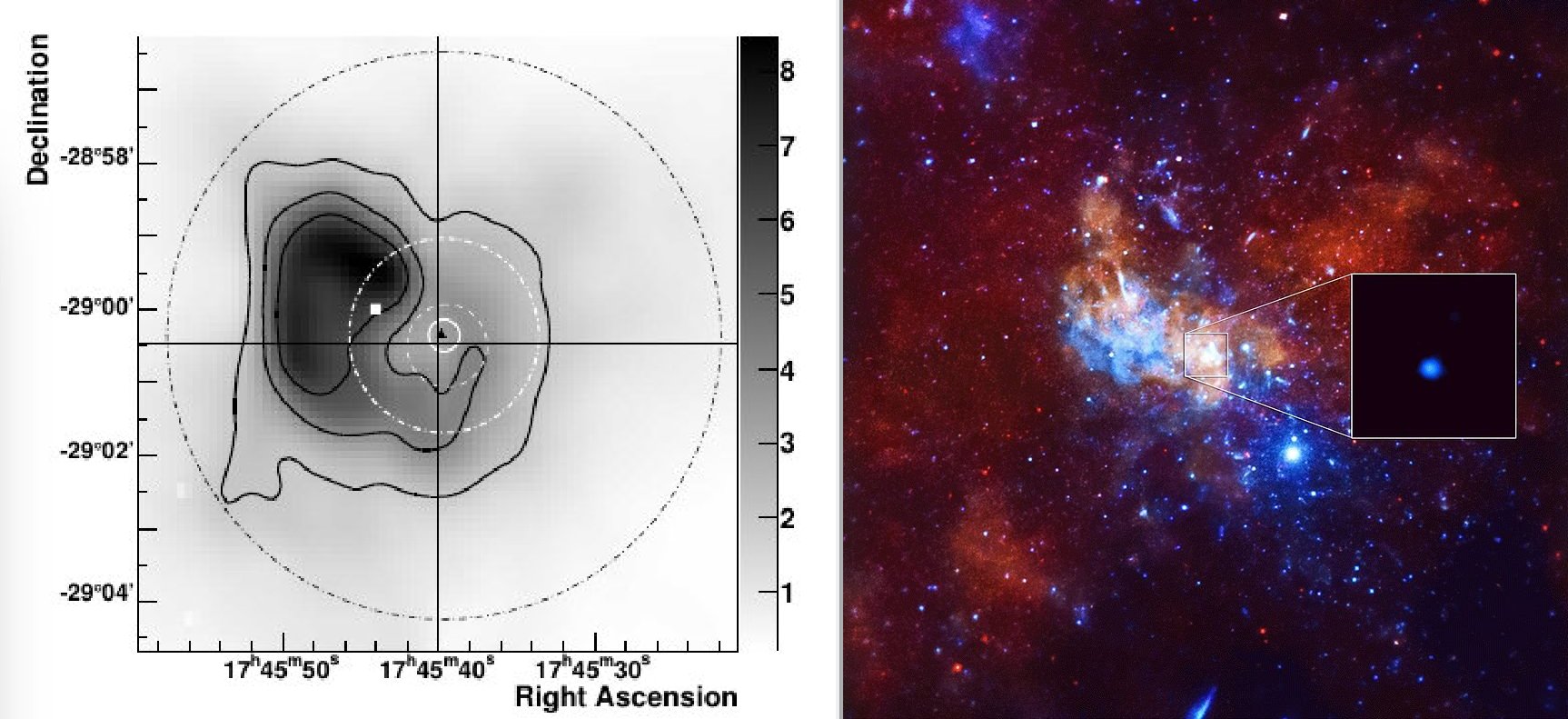
The spectrum of the gamma-ray emission in the TeV band derived from a circular region with a radius of 0.1 degree centered around Sagittarius A* can mathematically be described as a power-law with an exponential cut-off of the form dN/dE ∝ E−Γ0 · e(−E/Ecut) with a so-called photon spectral index Γ0= 2.14 ± 0.02stat ± 0.10syst and a cut-off energy of Ecut = 10.7 ± 2.0stat ± 2.1systTeV [7]. While derived with very good precision over a broad energy range, the spectral shape does not provide a unique clue on the source of the emission (see also H.E.S.S. SOM 12/2009). It is remarkable that the average energy flux in the gamma-ray band matches the average flux at lower energies.
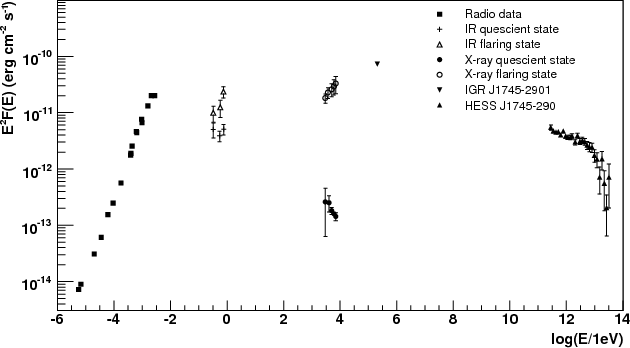
X-ray and infrared observations have revealed rapid variations form Sagittarius A*. While an association of these flares to the supermassive Black Hole Sagittarius A* was always considered plausible, the most convincing evidence for such an association emerged from the recent studies of the GRAVITY team [9]. When charting the motions of stars in the vicinity of Sagittarius A*, observations obtained with the GRAVITY instrument caught an infrared flare with sufficient precision to trace the proper motion of the flaring source. The movement in the vicinity of Sagittarius A* occurs at 30% of the speed of light, clearly associating the flare to material close to the horizon of the supermassive Black Hole. The radiation emitted from any source moving at such velocities will be amplified according to the laws of special relativity. It is tempting to associate these changes with gamma-ray variability recorded in Quasars, which is generally thought to be due to relativistic amplification, too. So far, however, no significant spectral variability of HESS J 1745-290 was found in the TeV band - neither on short nor on long time scales [8]. The most promising searches of short-term gamma-ray variations are those that can be conducted simultaneous to X-ray or infrared observations. The recent achievements in studies of Sagittarius A* suggest a clear path for further searches for gamma-ray variability conducted together with X-ray or infrared measurements. Ideally, any such observations are accompanied by high-resolution radio observations, which also hold the promise of pinpointing the sites of non-thermal radiation with great precision. The HESS collaboration had intended to team up with radio interferometric studies of the Galactic Center source this year, but Covid-19 prevented the execution of the radio observations. We are looking forward to new opportunities in the future.
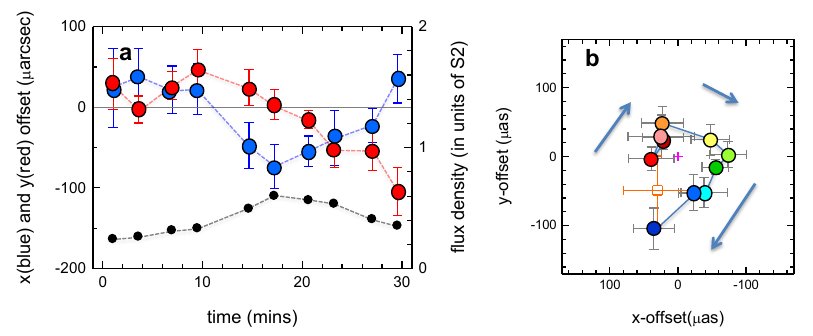
Irrespective of variability, the gamma-ray emission of HESS J1745-290 could also be related to non-thermal processes in the magnetosphere of the supermassive black hole [10], due to proton-proton-interactions of escaping high energy protons in the surrounding dense gas environment [11] (see also H.E.S.S. SOM 04/2016), or due to the acceleration of electrons in shocks of a wind emerging from the innermost parts of the accretion disk around the black hole [12].
References:
[1]
https://www.nobelprize.org/prizes/physics/2020/press-release/
[2]
Kosack, K., Badran, H.M., Bond, H.I., et al. 2004, ApJ, 608, L97.
[3]
Aharonian, et al. (HESS collaboration) 2004, Astron. Astrophys., 425, L13.
[4]
Abdalla et al. (HESS Collaboration), 2018, PRL, 120, 201101.
Abdalla et al. (HESS Collaboration), 2016, PRL, 117, 151302.
Abdalla et al. (HESS Collaboration), 2016, PRL, 117, 111301.
Abramowski et al. (HESS Collaboration), 2011, PRL, 106, 161301.
Abramowski et al. (HESS Collaboration), 2013, PRL, 110, 041301.
Abramowski et al. (HESS Collaboration), 2015, PRL, 114, 081301.
Aharonian et al. (HESS Collaboration), 2006, PRL, 97, 221102.
[5]
Acero et al. (HESS Collaboration), 2010, MNRAS, 402, 1877.
[6]
https://www.chandra.si.edu/photo/2015/sgra/
[7]
Abramowski et al. (HESS Collaboration), 2016, Nature, 531, 476.
[8]
Aharonian et al. (HESS Collaboration), 2009, Astron. Astrophys., 503, 817.
[9]
Abuter et al. (GRAVITY Collaboration), 2018, Astron. Astrophys., 618, L10.
[10]
Aharonian & Neronov, 2005, ApJ, 619, 306.
[11]
Aharonian & Neronov, 2005b, Ap&SS, 300 255.
Liu et al., 2006, ApJ, 647, 1099.
Ballantyne et al., 2007, ApJ, 657, L13.
[12]
Atoyan & Dermer, 2004, ApJ, 617, L123.