Extended emission around the Geminga pulsar
April 2020
Geminga (Gemini gamma-ray source) was discovered in the early 1970s with the SAS-2 satellite and remained an unidentified gamma-ray source for a very long time. It is now known as a radio-quiet gamma-ray pulsar, among the first gamma-ray pulsars to be detected by EGRET, located comparatively nearby to Earth at only 250 pc (or 815 lightyears) distance away ([1]). Compared to the majority of pulsars with associated gamma-ray emission it is considerably older and its spin-down luminosity – the amount of energy released per second – is considerably lower. However, this is more than compensated for by its proximity – as a result gamma-ray emission of Geminga and its surrounding wind are bright and the nebula is highly extended. Pulsars are copious sources of electrons and positrons; these expand into the surrounding environment within a pulsar wind nebula. In older systems such as Geminga, however, the pulsar wind nebula becomes disrupted, the particles are no longer confined and can diffuse out into the interstellar medium, forming a leptonic halo. Gamma-ray emission is produced by Inverse Compton scattering of these particles on the ambient radiation fields. In 2017, the HAWC experiment confirmed the Milagro detection of extended gamma-ray emission around the Geminga pulsar, on angular scales ≥2 degrees; both HAWC and Milagro are water Cherenkov based detectors with a wide field-of-view ([2]). Imaging Atmospheric Cherenkov Telescopes (IACTs) such as HESS, however, have a smaller field-of-view and detection of diffuse emission extended over comparable angular scales is challenging. At lower gamma-ray energies the nebula is expected to be even more extended and the identification and morphological analyses of the nebula are severly affected by source confusion and particulars of interstellar emission models ([3]).
Morphological studies of low surface brightness sources with angular sizes comparable to the field-of-view of any instrument require precise knowledge of the angular acceptance and reliable background estimations. A recent HAWC-HESS comparison study investigated the effects of different background estimation methods and analysis techniques typically used by the different detector technologies ([4]). In the so-called ring background approach, a common method for atmospheric Cherenkov telescope arrays, levels of background emission are estimated from regions at fixed offset from the test position within the field-of-view, with known regions of significant emission excluded ([5]). In the so-called field-of-view (FoV) background approach, a model for the gamma-ray acceptance behaviour of the instrument is used to estimate the background, assuming radial symmetry. In this comparison study, it was found that by increasing the angular sizes probed in the ring background approach and/or by using the FoV background approach to analyse HESS data, the region of the Galactic plane visible to both HESS and HAWC became more compatible. Therefore, we applied a similar analysis technique to the Geminga region.
Fourteen hours of observation data was taken on Geminga. While the detection of extended emission had proven elusive using conventional analyses, applying the lessons learned from the HAWC-HESS comparison study enabled extended TeV gamma-ray emission around the Geminga pulsar to be detected with both the ring background and FoV background approaches, as shown in figure 1. Within one degree radius of the pulsar position, extended emission is detected with a significance of almost 11 sigma.
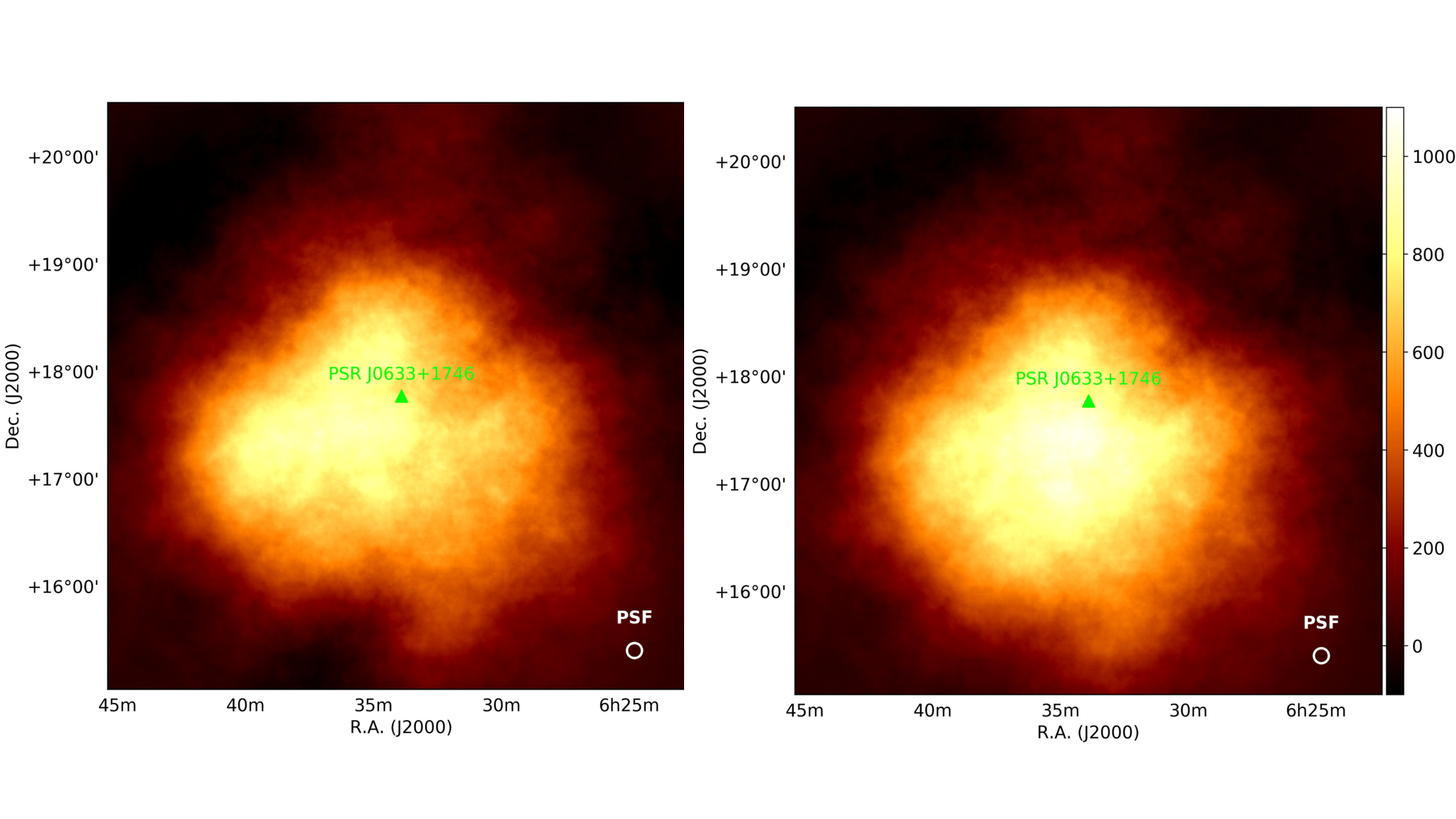
The integration region radius is the radial distance from the pulsar out to which emission is included in the significance calculation. As the significance continues to increase up to one degree radius without any signs that the curve is flattening (figure 2), we conclude that the true extent of the emission is greater than one degree radius. For the rather nearby pulsar Geminga, such angular scales correspond to a physical size of about 10 parsec. The so called 'off regions' of the ring background estimate are still within the angular extent probed by the HAWC experiment (figure 3). While a comparison of the two methods is restricted to such angular scales, the five degree field-of-view of HESS enables studies out to larger angular distances than those typically probed by atmospheric Cherenkov Telescopes using the ring background approach.
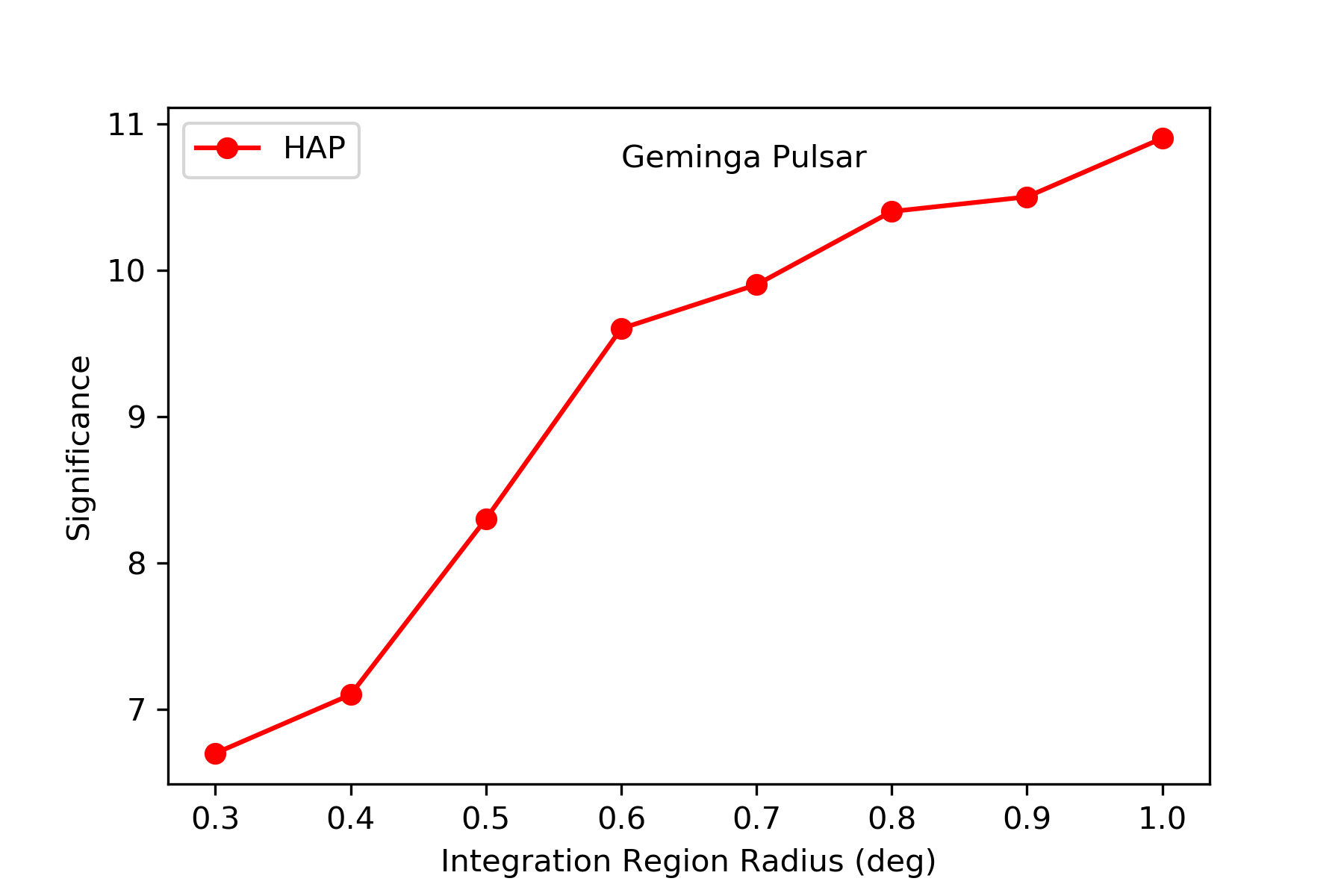
Figure 3 shows the profile of gamma-ray emission obtained from the HAWC experiment [2]. The regions used by HESS as the ON (signal) and OFF (background) regions for the ring background analysis are indicated by shaded regions. The ring background approach is therefore a measurement of how significant the ON region is relative to the OFF region, i.e. of the difference in significance. From the HAWC emission profile, this approach should detect approximately 35% of the total flux in the energy band covered by HAWC.
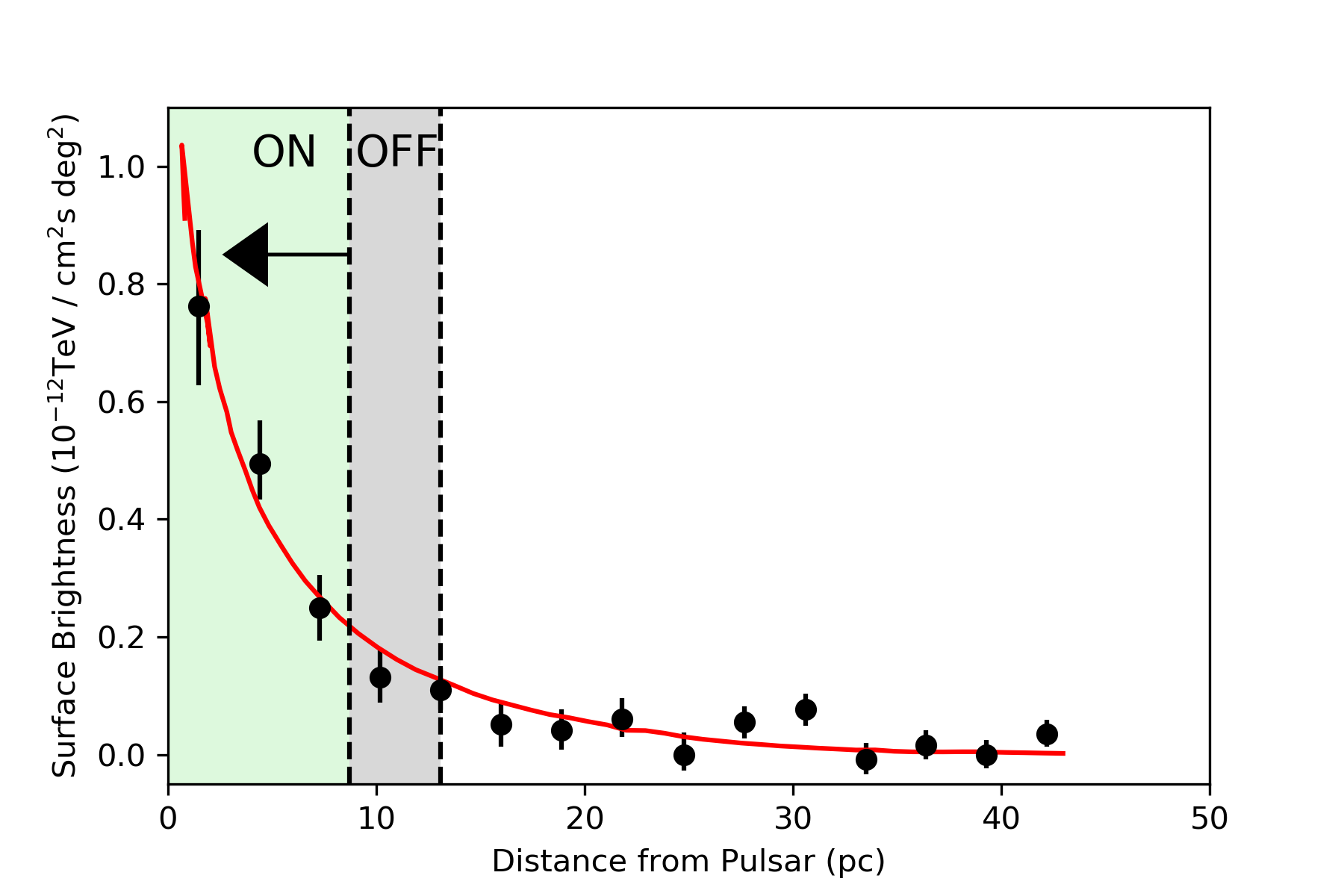
This detection demonstrates that highly extended emission can be detected by atmospheric Cherenkov telescopes despite their limited field-of-view. While such detections are challenging, the combination of source proximity and the (comparatively) high angular resolution allow studies to be conducted of the diffuse emission on small physical scales and changes of the overall morphology in different energy bands. The high proper motion of Geminga provides a clock for morphological studies with sufficient angular resolution - as is indicated by the spatial offset between the centroid and the pulsar position shown in figure 1.
References:
[1] Thompson et al, ApJ Supp., 101, 259-286 (1995)
[2]
HAWC collaboration, Science, 358, 911-914 (2017)
[3]
Di Mauro, Manconi and Donato, Phys. Rev. D 100, 123015 (2019)
[4] https://www.mpi-hd.mpg.de/hfm/HESS/pages/home/som/2020/02/
[5]
Berge, Funk and Hinton, A&A, 466, 1219-1229 (2006)