Core-collapse supernovae: promising candidates for PeV cosmic-ray acceleration?
July 2019
The quest of the sources of cosmic-ray (CR) particles is a hot topic in high energy astrophysics. So far, Supernova Remnants (SNRs) have been proven to be the good candidates concerning CR particles with an energy up to 1014 eV. The remnant is created after the explosion a massive star, an event known as a Supernova (SN). Supernovae are among the most energetic phenomena known so far, releasing an remarkable high energy of ~1051 erg in the interstellar medium. A shock wave is created, and relativistic CR electrons, protons, and nuclei are accelerated at the SNR shock front through a complex and efficient mechanism that can last thousands of years.
CR sources can only be identified indirectly through the electromagnetic and neutrino emission caused by interactions of CR particles with local gas and radiation. In this respect, gamma-ray observations have proven to be an invaluable tool, as very high energy (VHE; 50 GeV < E < 100 TeV) gamma-ray emission is uniquely associated with the presence of highly energetic CR particles, either produced by the decay of a neutral pion (the so-called "hadronic scenario"), or by the scattering of high-energy electrons on low energy photon, known as the Inverse Compton process ("leptonic scenario"). Surprisingly, the direct observation of gamma rays produced from protons and nuclei through neutral pion decay have been observed in rather old SNRs, with an age of several thousands of years [1], whereas young ones, like the ~300 year old gamma-ray emitter Cassiopeia A, exhibit gamma-ray spectra with relatively low energy cut-off at 1 to 10 TeV. Particularly beyond some feature of the CR spectrum called the "knee", when the CR particles are reaching 3x1015 eV (=3 PeV), the CR sources have not been firmly identified yet, even if, recently, a blazar has been identified as a powerful cosmic ray accelerator (see SOM 2018/08).
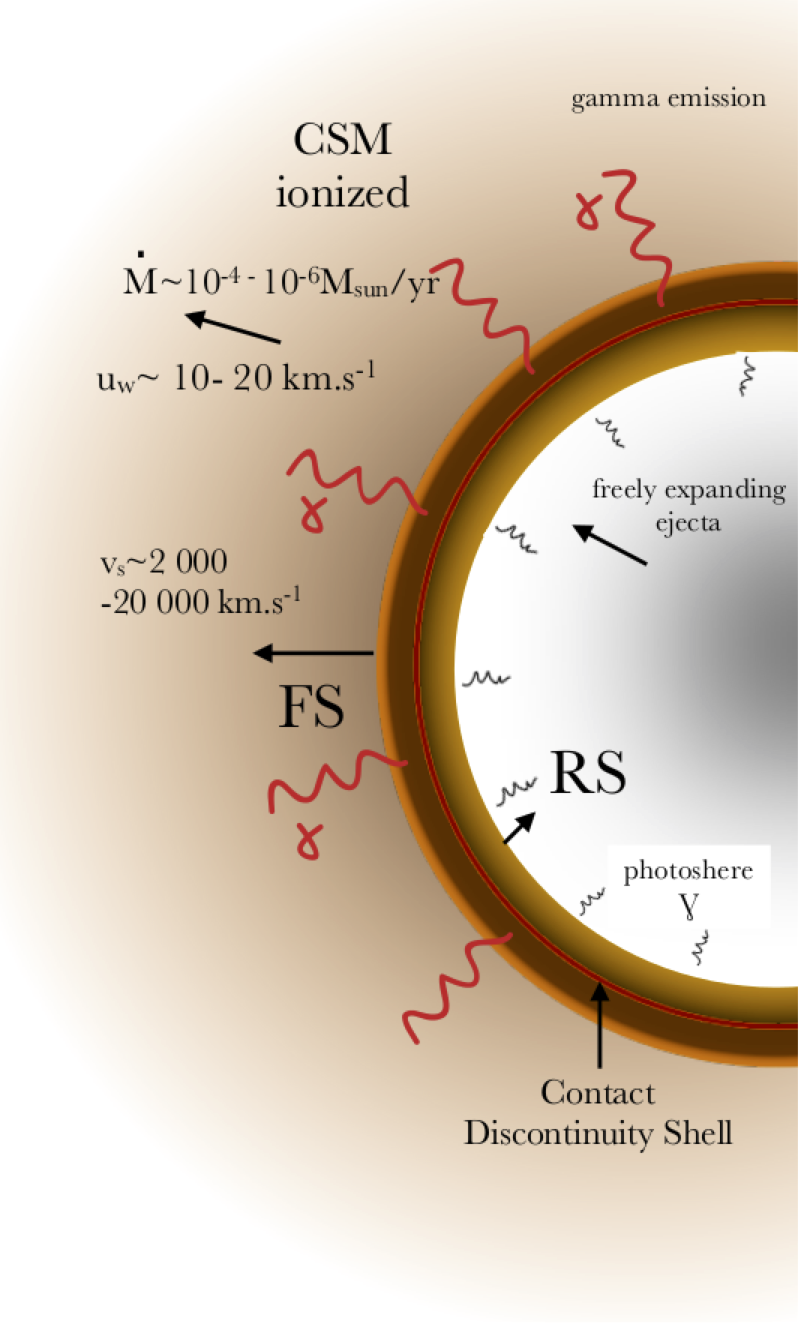
But how can an efficient acceleration mechanism take place so early?
The models predict that, when the SN shock propagates in a very dense CSM, a collision-less shock can be formed within few days: the high shock velocity and the CSM ionisation allow for particle acceleration driving the growth of plasma instabilities, which in turn facilitate particle acceleration: iteration of the process leads to a fast and high magnetic field amplification. Such a high magnetic field is indeed necessary to accelerate particles to VHE at an early stage (e.g [2]-[4]).
High magnetic field of the order of Gauss are recorded in radio SNe, instead of the 10 to 100 μG measured in young SNRs. For example, concerning the nearby Type IIb SN 1993J, a magnetic field as high as 100 G and shock speeds as high as 20,000 km s-1 have been estimated [5]. Given these estimates and time scales for acceleration to PeV energies, there are good reasons to think that SN 1993J-like candidates can accelerate CR hadrons up to PeV energies within days to a few weeks (see [6], and references therein). Some relevant quantities for PeV acceleration are sketched in Figure 1: the dense CSM is created by the progenitor wind which shall exhibit an high mass-loss rate together with a low wind velocity.
In that perspective, we searched for gamma-ray emission from ten catalogued cc-SNe that occurred in nearby galaxies and were observed with H.E.S.S. within a year of the SN event. Nine cc-SNe were observed serendipitously between December 2003 and December 2014, with exposure times ranging from 1.4 hours to 53 hours. The selected sample consist of 9 Type IIP SNe with distances spanning ~4 to ~53 Mpc. In addition, we observed the type IIb candidate SN 2016adj in Centaurus A (at 3.8 Mpc distance) as a target of opportunity in February 2016 for 13 hours.
No significant gamma-ray emission has been detected for any of the objects, and upper limits (ULs) on the >1 TeV gamma-ray flux of the order of ~1013 cm-2s-1 are established. We translated these constraints into luminosity values above the energy threshold, spanning a range from ~2x1039 erg s-1 to ~1x1042 erg s-1. Note that these ULs correspond to gamma-ray fluences within a year of ~6x1046 erg to ~3x1049 erg, corresponding to 0.006 to 3 percent of the canonical SN explosion energy of 1051 erg. The detailed results can be found in [8].
We could also convert these measurements into constraints on the environment of the SNe. For that purpose, we used the model derived in [7] concerning gamma-ray emission of hadronic origin of young SNRs. Using the equation 9 of [7], we could put limits on the mass-loss rates, which determine the CSM density (for an assumed wind velocity of 10 km s-1). We calculated mass-loss rate ULs spanning from ~2.5x10-5 M☉yr-1 up to ~1.6x10-3 M☉yr-1. These values can be compared to the estimated mass-loss rate of SN 1993J of 3.5 10-5 M☉yr-1 [5]. These results are consistent with the commonly predicted mass-loss rates for type IIP SN progenitors, which are of order 10-6-10-4 M☉yr-1.
This non detection can be related to similar studies, given that SNe have not been detected in HE gamma rays either so far [9,10]. However this result does not disprove that SNe can accelerate CRs, as the CSM densities may be too low for detectable gamma-ray emission. We may have not observed the best candidates. Using the same model, Figure 3 shows that a type IIP SNe with a mass loss rate of 10-4 M☉yr-1, occurring at a distance of 10 Mpc, shall be detectable with H.E.S.S. 20 days after outburst. CTA could detect a type IIP SNe with a mass loss rate of 10-4 M☉yr-1, occurring at 30 Mpc. SNe within 10 Mpc occur regularly, and should be followed up with gamma-ray observations. However SNe with such a dense CSM may not be common: in [8], we predict that among all types, SNe with a mass loss rate above 10-4 M☉yr-1 should occur within a radius of 10 Mpc at a rate of one per year. We are confident that Cherenkov telescopes, and in particular CTA, should observe gamma-ray emission from SNe within a few weeks after the outburst in a near future.
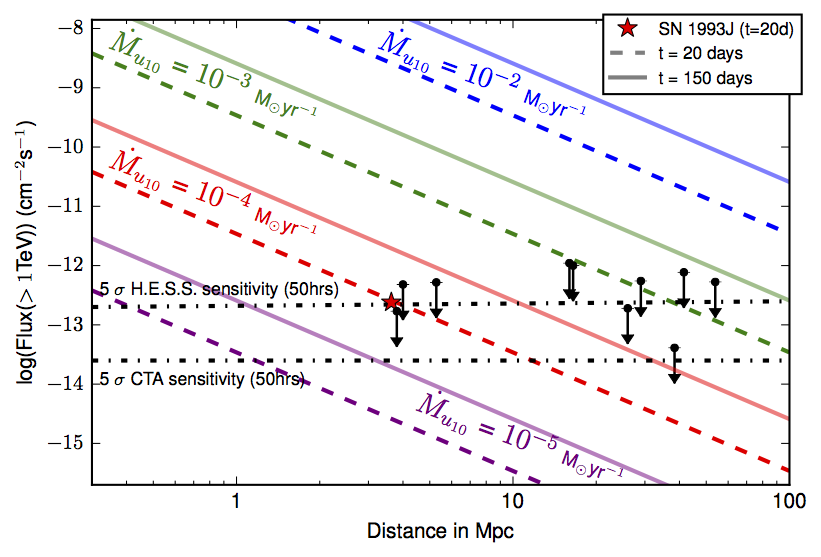
References:
[1] M. Ackermann et al., "Detection of the Characteristic Pion-Decay Signature in Supernova Remnants", Science 339 (2013) 807-811
[2] V.S. Ptuskin and V.N. Zirakashvili, "On the spectrum of high-energy cosmic rays produced by supernova remnants in the presence of strong cosmic-ray streaming instability and wave dissipation", Astronomy & Astrophysics 429 (2005) 755-765.
[3] M. Cardillo, E. Amato and P. Blasi, "On the cosmic ray spectrum from type II supernovae expanding in their red giant presupernova wind", Astroparticle Physics 69 (2015) 1-10
[4] A. Marcowith et al., "Core-collapse supernovae as cosmic ray sources", Mon Not R Astron Soc 479 (2018) 4470-4485
[5] V. Tatischeff, "Radio emission and nonlinear diffusive shock acceleration of cosmic rays in the supernova SN 1993J", Astronomy & Astrophysics 499 (2009) 191-213
[6] A. Marcowith et al., "Cosmic-ray acceleration and gamma-ray signals from radio supernovae", Nuclear Physics B - Proceedings Supplements 256-257 (2014) 94-100
[7] V.V. Dwarkadas, "Exploring the gamma-ray emissivity of young supernova remnants - I. Hadronic emission", Mon Not R Astron Soc 434 (2013) 3368-3377
[8] A. Abdalla et al. (H.E.S.S. Collaboration), "Upper Limits on Very-High-Energy Gamma-ray Emission from Core-Collapse Supernovae Observed with H.E.S.S.", Astronomy & Astrophysics 626 (2019) A57
[9] N. Renault-Tinacci et al., "Search for gamma-ray emission from super-luminous supernovae with the Fermi-LAT", Astronomy & Astrophysics 611 (2018) A45
[10] M. Ackermann et al., "Search for Early Gamma-ray Production in Supernovae Located in a Dense Circumstellar Medium with the Fermi LAT", The Astrophysical Journal 807 (2015) 169
[11] N. Smith et al., "Precursor Eruptions and the Optically Thick Regime of Extremely Luminous Type IIn Supernovae", Astrophysical Journal 686 (2008) 467-484