The Blazar 1ES 1101-232 and the Gamma Ray Horizon
December 2005
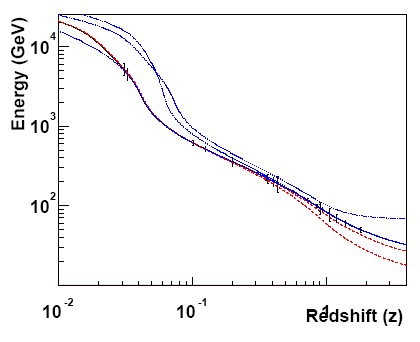
Cherenkov telescopes such as H.E.S.S. use very high energy (VHE) gamma rays to explore the Universe. But how far can these gamma rays travel, and which fraction of the Universe can be viewed? Interstellar gas can hardly stop VHE gamma rays; they easily penetrate a few g/cm2 of matter, much more than is found in interstellar gas clouds. However, surprising as it may sound at first, starlight can stop gamma rays. A gamma ray of a few TeV energy has enough energy to create, upon "colliding" with a starlight photon, an electron-positron pair, resulting in the absorption of the gamma ray (e.g. Gould and Schreder 1966, Jelley 1966, Stecker et al. 1992). The higher the energy of the gamma ray, the lower can be the energy (or frequency) of the photon. For gamma rays of tens of TeV, even far-infrared photons contribute to the absorption. Therefore, the degree of absorption tends to increase with gamma-ray energy. The figure on top illustrates how far (in redshift) a gamma ray of a given energy can typically travel; at TeV energies, range appears limited to redshifts around 0.1.
What at first sounds like a limitation - the absorption of TeV gamma rays - can be turned into an advantage. For a source at a given distance (or redshift), gamma ray spectra should show a cutoff or should at least steepen around the energy displayed in the top figure. The degree of absorption, and hence the precise energy of the cutoff, depends on the intensity and spectrum of light in intergalactic space and hence allows measuring this intensity. This intensity of starlight is of significant cosmological interest, since it represents the light emission of galaxies summed over the entire history of galaxy formation since the Big Bang (e.g. Kashlinsky 2005). Direct measurements (for a review see Hauser and Dwek 2001) on or near Earth are very difficult, because of overwhelming amounts of foreground light originating in the solar system or in our own Galaxy. A heavily debated peak in the light spectrum at wavelengths around 1-2 micrometers (Matsumoto et al. 2005), e.g., might be an indication of emission from the very first stars in the Universe ("Population III stars", e.g. Salvaterra and Ferrara 2003, Madau and Silk 2005), but could also be a remaining foreground contamination.
VHE gamma ray spectra of distant blazars have been used by various authors to estimate the level of extragalactic background light (EBL) (see e.g. Dwek and Krennrich 2005 and refs. given there). The discovery by H.E.S.S. (Fig. 1) of the distant blazar 1ES 1101-232 as source of gamma rays with a spectrum extending up to several TeV allows most sensitive tests of the EBL level. 1ES 1101-232 was detected first as an X-ray and radio source, the name refers to the survey by the Einstein X-ray satellite. The redshift of the source was determined to z= 0.186 (Remillard et al. 1989, Falomo et al. 1994); it is currently the most distant AGN with a measured VHE gamma ray spectrum. For typical EBL levels assumed so far, gamma rays at 1 TeV should be suppressed by almost a factor 1000 due to absorption (Fig. 2), whereas gamma rays at 0.1 TeV show very little absorption, since they do not have enough energy for pair production unless interacting with photons in the extreme UV. The absorption therefore makes energy spectra steeper. However, the measured spectrum (Fig. 3), described as dN/dE ~ E-Gamma, with a spectral index Gamma = 2.9, is not nearly as steep as expected, given that models for gamma ray emission allow spectra at the source with an index Gamma not less than 1.5. Absorption due to canonical EBL should then result in very steep measured spectra, with Gamma of 4.5 or more. To reproduce the spectrum measured by H.E.S.S., the EBL intensity can be not more than 1/2 of the level commonly assumed so far. Interestingly, this reduced EBL intensity almost coincides with the starlight emitted by the galaxies which were counted in galaxy surveys, implying that almost all sources of the EBL have been seen in these surveys. The H.E.S.S. results also show that the Universe is more than transparent to gamma rays than previously assumed.
References:
Resolving the Extragalactic Background Light with gamma-ray spectra from distant blazars, F. Aharonian et al., astro-ph/0508073 (2005)
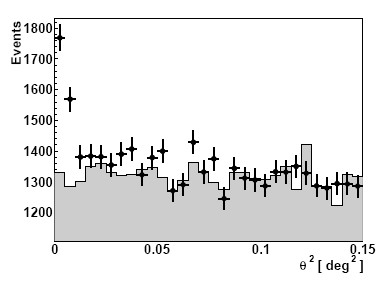
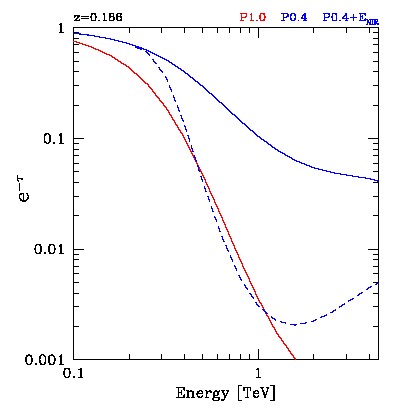
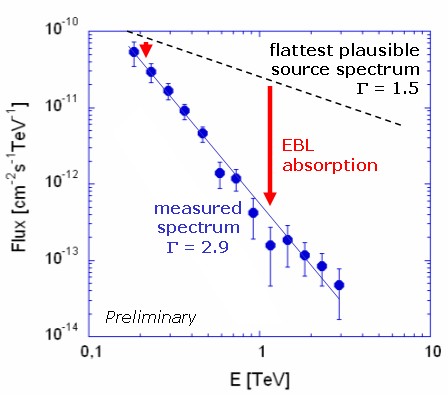