News Archive 2019
The KATRIN experiment narrows the mass of neutrinos down further
In 1930 Wolfgang Pauli postulated a new neutral particle to explain the β-decay. It was named "neutrino" by Enrico Fermi in 1933 and in
1956 the existence of neutrinos was experimentally proved. Apart from photons, neutrinos are the most abundant elementary particles in
the universe. About hundred trillion neutrinos are traversing every human per second.
Neutrinos were long believed to be massless, but experiments between 1997 and 2002 showed that the neutrinos change their flavour
(electron, muon, or tau neutrino) periodically. According to theory, this so called neutrino oscillation can only occur if neutrinos
have mass.
So far, only upper limits of the neutrino mass could be determined, confirming it to be tiny but not zero, contrary to the prediction of
the current Standard Model of particle physics. Thus, neutrinos and their small non-zero masses play a prominent role in the evolution of
large-scale structures in the cosmos, as well as in the world of elementary particles, where their small mass scale points to new physics
beyond known theories.
Precision measurements of the kinematics of weak interactions represent the only model independent approach to determine the absolute
scale of neutrino masses. The β-decay of 3H (tritium) to 3He is the most promising candidate for the direct mass determination of the
neutrino. A neutrino mass larger than zero modifies the shape of the energy spectrum of the emitted β-electron near the high-energy endpoint.
The KArlsruhe TRItium Neutrino (KATRIN) experiment
located at the Karlsruhe Institute of Technology (KIT) has been designed to measure
the mass of the electron antineutrino directly with an unprecedented sensitivity of 0.2 eV/c2.
In a recent article published in Physical Review Letters the KATRIN collaboration, formed by 20 institutions from 7 countries, presents the results of a first four-week science run in spring 2019. During this measurement campaign, high statistics energy spectra of electrons from tritium β-decay were collected. Subsequently, three international analysis teams worked separately on extracting the first KATRIN neutrino mass result. No team had the complete information to prematurely deduce the neutrino mass result before completion of the final analysis step. To coordinate their final steps, the analysts met for a week-long workshop at KIT in mid-July 2019. Their analysis programs independently yielded identical results. They limit the absolute mass scale of neutrinos to a value of less than 1.1 electron-volt (eV)/c2 at 90% confidence. Thus, a half of millions of the neutrinos weigh less than one electron, the second lightest elementary particle.
With the now established world-leading upper limit on the neutrino mass, the KATRIN experiment has taken its first successful step in elucidating unknown properties of neutrinos. The KATRIN collaboration looks forward to further significant improvements of the neutrino mass sensitivity and in the search for novel effects beyond the Standard Model of Particle Physics.
Please read more in the article ... >
The article has been selected for a Viewpoint in Physics. Please read also the
viewpoint on the article by
R. Brugnera.
Further press releases:
First experimental constraint on the coupling strength of antimatter with dark matter candidate
Humans have always been fascinated by the observation of the starry sky und tried to find regularities and fundamental laws in order to understand and predict the observable phenomena. It was not until the 20th century, however, that scientists realized that the stars and the other visible astronomical objects are made of ordinary matter and thus obey the already well-known laws of physics. In the early 1930s, the astronomers Jan Hendrik Oort and Fritz Zwicky postulated the existence of unvisible ("dark") ordinary matter to explain several new astronomical observations. But only from the 1960s onwards, it became clear by analyses of the dynamics of galaxies, that there has to be about five times more so-called "dark matter" in our Universe in addition to the well-known visible "baryonic" matter. Moreover, to explain the observed accelerated expansion of the Universe, since the 1990s the physicists assume that about 70% of the energy density of the Universe consists of so-called "dark energy".
The microscopic properties of "dark matter" and "dark energy" remain unknown. Many physicists assume that dark matter particles are
electrically neutral and are only weakly interacting with ordinary matter. One dark-matter candidate are the so-called axions, which
are light and spinless bosons that were already postulated as hypothetical particles in 1977. Originally, to explain the experimentally
observed complete symmetry of the strong interaction, which seems unnatural considering the symmetry violation involved in the weak
interaction.
Neither axions nor other dark-matter candidate particles could be experimentally detected so far. Not only the nature of
dark matter and dark energy is not yet understood. Even the matter-antimatter imbalance of the ordinary visible matter, that accounts
for about 5% of the total energy density of the Universe, still lacks any consistent explanation.
At present, we have no direct information on the strength of the interaction between axions and antimatter. According to the fundamental and so far always experimentally confirmed charge-, parity- and time-reversal (CPT) invariance of the Standard Model, particles and antiparticles have equal coupling strengths. For example, if antiprotons couple stronger to axions or axion-like particles (ALPs) than protons, such an asymmetric CPT-odd (i.e. charge-reversal) coupling could provide a link between dark matter and the matter-antimatter imbalance in the Universe.
In a recent article published in "Nature" C. Smorra, the
BASE collaboration
and the Budker group
from the Helmholtz Institute Mainz
report on the direct experimental search for
interactions between antimatter and dark matter. The measurement was performed at the
Antiproton Decelerator (AD)
of CERN, Geneva, utilizing
a novel two-particle spectroscopy method in an advanced cryogenic multi-Penning trap system. More information about the used novel
spectroscopy method can be found in our
news of 18.10.17 and the corresponding "Nature" article
(Nature 550, 371–374 (2017)
). The result
reported in the present article searches for periodic changes of the antiproton spin precession frequency as signature for the
axion-antiproton interaction. The measurements allowed setting considerable first constraints on the possible
strength of the interaction between ultralight axion-like particles with antiprotons, which are five orders of magnitude more sensitive
than astrophysical limits.
According to theory, the interaction of fermions, e.g. antiprotons, with axions causes a spin precession analogous to the known precession
of the particle spin in an external magnetic field (Larmor precession). These spin-precession effects from ultralight axions have a
characteristic frequency governed by the mass of the underlying particle. Thus, a potential coupling of antiprotons and axions can be
detected by a Fourier analysis of the spin-flip resonance data acquired with a single antiproton in a Penning trap. The researchers found
no significant indication of a periodic interaction of the antiproton spin with the axion field.
The performed data analysis constrained
the axion-antiproton interaction parameter to values greater than 0.6 GeV.
In the future, improved limits will be
provided by more precise measurements of the antiproton spin precession frequency, and similar searches for interaction effects with axions
can be performed for other antiparticles, such as positrons and anti-muons.
Please read more in the "Nature" article
and the associated article in News & Views
.
Further information also in the press release of the MPIK .
Further press releases:
- Press Release Johannes Gutenberg University Mainz
(idw
)
- BASE News
- CERN News
- EurekAlert! Science News: University Mainz
| RIKEN
- Gizmodo Science News
- New Atlas Science News
- Phys.org Physics News
- ScienceDaily Science News
- scinexx News
- SpaceDaily News
- Tech Explorist Science News
- Technology Org Physics News
- VICE Tech News
- WISSEN.NEWZS.de
- cosmos-indirekt.de - News aus der Physik
Improvement of the experimental g factor of lithiumlike silicon challenges bound-state QED
The measurement of the magnetic moment of charged elementary particles and simple systems allows for stringent tests of fundamental
physical theories. The g factor describes the ratio of the magnetic moment of a charged particle and the total angular momentum.
Advanced Penning-trap setups are perfect tools for high-precision determination of g factors of stored highly charged ions.
The comparison of the experimental results with elaborate theoretical g-factor calculations provides the most stringent test of
bound-state quantum electrodynamics (BS-QED) in the presence of a magnetic field. Moreover, precise g-factor measurements allow
for the determination of the electron mass, which is a central quantity in the Standard Model of particle physics
(see our news of 20.02.14). The accurate values of fundamental constants, e.g. the fine structure constant α, depend on the
precise knowledge of the electron mass.
Nuclear effects, e.g. the nuclear recoil effect, set the ultimate limits of the theoretical g-factor accuracy. In 2016, the most
stringent test of the nuclear recoil effect in a magnetic field was performed (see our
news of 18.01.16). The theoretical accuracy limits
for g-factor and fine structure constant α calculations can be overcome by studying specific differences of the g factors of hydrogenlike
and lithiumlike or boronlike ions. In 2013, the g factor of lithiumlike silicon 28Si11+ has been determined with a relative
experimental uncertainty of 1.1·10-9 (see our
news of 16.01.13) and a corresponding relative theoretical uncertainty of
3·10-9. In 2016, the g factors of the lithiumlike calcium isotopes 40Ca17+ and 48Ca17+ have been determined with two-times
smaller uncertainty (see our news of 18.01.16) and a corresponding relative theoretical uncertainty of
6·10-9.
The established agreement between experiment and theory for the g factors of lithiumlike silicon and calcium ions manifests the most
stringent test of the many-electron bound-state quantum electrodynamics (BS-QED) effects in the presence of a magnetic field.
In a recent article published in Physical Review Letters D. A. Glazov et al. present simultaneous experimental and theoretical
improvements of the g factor of lithiumlike silicon. The g-factor measurement was performed at Johannes Gutenberg University Mainz,
Germany, in a double Penning-trap system consisting of a precision trap (PT) and a spatially separated analysis trap (AT). The
g-factor determination of lithiumlike silicon is based on the measurement of the frequency ratio Γ ≡ νL/νc of the Larmor precession
frequency (νL) and the cyclotron frequency (νc) of a single stored 28Si11+ ion inside the Penning trap.
The cyclotron frequency νc can be determined from the three independent harmonic oscillations νz, ν+ and ν- of the ion motion in
the precision trap using the Brown-Gabrielse invariance relation: νc = (ν+2 + νz2 + ν-2)1/2. The axial motion νz can be detected via
the image currents that the silicon ion induces onto the trap electrodes. The modified cyclotron frequency ν+ and the magnetron
frequency ν- are measured by coupling the axial and the radial modes via suitable radiofrequency excitations using radio frequency
drives on the motional sidebands at ν+-νz and νz+ν-. For the most important modified cyclotron mode the novel phase-sensitive
PnA (Pulse and Amplify) detection technique is used.
The determination of the Larmor precession frequency νL is based on the non-destructive detection of spin flips using the continuous
Stern-Gerlach effect in the analysis trap. For this purpose, in the AT the homogeneous 3.8 T magnetic field of the PT gets modified
to an inhomogeneous magnetic field. The strong magnetic inhomogeneity (magnetic bottle) couples the spin direction to the axial
oscillation frequency νz of the 28Si11+ ion. Thus, a spinflip causes a clearly detectable axial frequency jump Δνz of about 260 mHz.
From the determined frequency ratio Γ of the stored 28Si11+ ion an experimental g factor
gexp = 2.00088988845(14) of lithiumlike silicon with a relative uncertainty of 7.0·10-11 was derived. This is a 15-fold improvement
of the previous experimental g-factor value.
The corresponding theoretical efforts yielded a theoretical g factor of 28Si11+
gth = 2.0008898944(34) with a relative uncertainty of 1.7·10-9. This represents a 2-fold improvement of the previous
theoretical g-factor value. The theoretical uncertainty is largely determined by the estimation of the presently unknown contributions
of the two-loop many-electron diagrams of the BS-QED. The difference between gth and gexp is 1.7 times larger than this uncertainty
which may indicate that these contributions exceed the present estimations.
The new experimental g-factor value is accurate enough to validate the non-trivial parts of the many-electron two-loop BS-QED
contributions on a few percent level as soon as they are calculated.
Please read more in the article ... >
Application of a novel detecting scheme for optical transitions in highly charged ions with ALPHATRAP
Highly charged ions (HCI) enable very important and unique tests of fundamental theories. The electrons in HCI experience very
strong electromagnetic fields and are thus predestined for testing bound-state quantum electrodynamics (QED) calculations in
extreme conditions as well as relativistic many-body interactions and nuclear effects. Furthermore, principal transitions in
HCI can be shifted to the keV range and fine structure or hyperfine structure transitions become accessible for laser
spectroscopy.
The commonly used devices to produce and store HCI typically feature conditions that hinder reaching high spectroscopic precision,
such as high temperatures in the case of an EBIT and high kinetic energies in storage rings. While in some cases traditional
fluorescence spectroscopy can be employed, in most cases the electric-dipole forbidden transitions have long lifetimes and
consequently prohibitively low photon yields.
In a recent article published in Physical Review Letters A. Egl et al. present a novel technique that enables an efficient
search for narrow forbidden transitions as well as the precise spectroscopy of the respective long-lived states. In order
to demonstrate the new detecting method, a single 40Ar13+ ion was stored in the cryogenic Penning-trap system of ALPHATRAP
at MPIK in Heidelberg. ALPHATRAP is a double-trap system consisting of a precision trap (PT) and an analysis trap (AT).
It allows to manipulate and spatially confine a single charged particle at low energies by a superposition of static electric and
magnetic fields. The ions are injected e.g. from the cryogenic Heidelberg Electron Beam Ion Trap (HD-EBIT) or a
Heidelberg Compact EBIT (HC-EBIT). Please read more about the ALPHATRAP experiment in the review article of S. Sturm et al. .
Laser spectroscopy was performed with ALPHATRAP on the 441 nm fine structure transition between the
2p 2P1/2 and 2P3/2 state in a single stored boronlike
argon ion 40Ar13+. For probing the fine structure transition by
irradiation with a collimated laser beam at 441 nm, the ion was transported to the PT, which has a highly homogeneous
magnetic field of 4.02 T. In the AT this field gets modified to an inhomogeneous magnetic field (magnetic bottle). This
couples the spin direction of the electron to the axial oscillation frequency νz of 40Ar13+ inside the trap. Thus,
depending on the orientation of the spin state of the electron the argon ion experiences a shift Δνz in
the axial frequency.
This measuring principle is called the continuous Stern-Gerlach Effect. It allows to read out and prepare the spin state
in the AT of ALPHATRAP by microwave irradiation. In the case of 40Ar13+ a change of
Δνz=±0.3 Hz after microwave irradiation indicates a transition between the two
2P1/2 ground state levels spin up or spin down.
The measurement procedure is as follows: ion preparation in one of the two Zeeman sublevels of the ground state in the AT - transport to the
PT where the ion is irradiated with a laser beam in order to excite the transition
ν1 (|J,mj〉 = |1/2,-1/2〉 → |3/2,+1/2〉) or ν2 (|1/2,+1/2〉 → |3/2,-1/2〉)
to the corresponding Zeeman sublevels of the excited state. After the decay of the excited state the ion is eventually pumped
into the other magnetic substate, which is far off resonant from the initial one and
therefore a dark state and can be detected by using the continuous Stern-Gerlach effect - shuttling back to the AT
where the spin state is analyzed and subsequently reinitialized into the initial spin state.
Repeating this procedure multiple times at different probe frequencies of the laser in a randomized order allows to deduce
a frequency dependent spin state transition probability and a resonance can be sampled.
The dipole-forbidden 2p 2P1/2 - 2P3/2 transition in the fine structure of 40Ar13+ could be determined with ALPHATRAP to a fractional uncertainty of 9.4·10-9. The new value of 679.216464(4)stat(5)sys THz is in reasonable agreement with the current best literature values and improves the uncertainty by a factor of 24.
The novel technique allows to sweep a large frequency range in order to find a transition which is not a priori known to sufficient precision. Combined with the possibility to store and cool arbitrary HCI in ALPHATRAP for virtually indefinite time spans, the method can be used to search very efficiently for strongly forbidden transitions in multi-electron HCI.
Please read more in the article ... >
Further information also in the press release of the MPIK .
CSR allows first investigation of the rotational dependence of dissociative recombination
At the beginning of the universe, several minutes after the Big Bang, only small nuclei existed, mainly hydrogen, deuterium
and helium, produced by so-called Big Bang nucleosynthesis at over 107 K. When temperatures lowered to about 2500 K, the
recombination of these nuclei with electrons led to first neutral atoms. Later, primordial molecules formed by radiative
association and charge-exchange reactions. The first molecule was the helium hydride ion (HeH+), formed by ionized hydrogen
and neutral helium atoms. Other primordial molecules are molecular hydrogen (H2), hydrogen deuteride (HD), and lithium
hydride (LiH).
The epoch of first star formation in the early universe was dominated by these simple primordial molecules. A gas cloud can cool
down to the low temperatures required for gravitational collapse and thus star formation by the process of radiative cooling.
Critical for this process is the molecular dipole moment, which is large for HeH+ and LiH. The much higher elemental abundance
of He relative to Li moves HeH+ into the focus of scientific interest. The abundance of molecular ions is often limited by
dissociative recombination with electrons, a process by which electrons destroy molecular ions. Uncertainties of the reaction
rate coefficients of such processes are today perceived as the only limitation on our understanding of primordial gas evolution.
At MPIK in Heidelberg, a novel cryogenic electrostatic storage ring CSR
(see our news of 18.05.16) allows to conduct
astrophysical laboratory experiments in order to understand molecular reactivity under primordial conditions.
In a recent article published in Science O. Novotny et al. report on accurate rate coefficient measurements of dissociative
recombination (DR) with HeH+ ions stored in the cryogenic storage ring CSR and a merged electron beam. All previous measurements
were performed for HeH+ dominantly in excited rotational levels at a rotational temperature of about 300 K. The measurement of
state-specific rate coefficients of DR in the CSR allowed for the first time to address the rotational dependence of DR. A dramatic
decrease of the electron recombination rates for the lowest rotational states of HeH+, compared to previous measurements at
room temperature, was found. The reduced destruction of cold HeH+ translates into an enhanced abundance of this important
primordial molecule at redshifts of first star and galaxy formation.
The new data show that the rotational excitation can make a dramatic difference in low temperature reaction rates of small molecules.
Please read more in the Science article:
Science Vol. 365, Issue 6454 (2019) pp. 676-679
| Full text
|
Reprint
Further information also in the press release of the MPIK
(idw
).
ALPHATRAP measures the g-factor of boron-like argon ions with highest precision
The g-factor describes the ratio of the magnetic moment of a charged particle and the total angular momentum. The g-factor of the bound electron permits high-precision tests of quantum electrodynamics (QED) in strong Coulomb fields. The currently most stringent test of QED in strong fields has been performed with hydrogenlike silicon (see our news of 08.07.11). Subsequently the so far confirmed QED theory has been used for the precise determination of the electron mass (see our news of 20.02.14). The most stringent test of the relativistic nuclear recoil effect in a magnetic field was performed by measuring the isotope shift of the g-factor of lithiumlike calcium (see our news of 18.01.16). Finally, relativistic many-electron correlations were investigated using lithiumlike silicon (see our news of 16.01.13).
The experimental determination of the g-factor of a boronlike ion allows, for the first time, for precision tests of QED involving a bound electron possessing orbital angular momentum, and for more stringent tests of many-electron correlations. Furthermore, such ions can also be used in the future for an independent determination of the fine-structure constant α.
In a recent article published in Physical Review Letters I. Arapoglou et al. present the first result of the ALPHATRAP experiment at the Max Planck Institute of Nuclear Physics. ALPHATRAP is a novel Penning-trap setup for high-precision determination of g-factors of stored highly charged ions. The ground-state g-factor of boronlike argon 40Ar13+ was measured with a fractional uncertainty of 1.4·10−9.
The ALPHATRAP double-trap system consists of a precision trap (PT) with a highly homogeneous magnetic field used
for high-precision spectroscopy and an analysis trap (AT) with an inhomogeneous magnetic field for spin-state
determination. The determination of the g-factor of a single 40Ar13+ with ALPHATRAP was based
on the measurement of the frequency ratio of the Larmor frequency (νL) and the cyclotron frequency
(νc).
The cyclotron frequency νc can be determined from the three harmonic components νz, ν+ und ν-
of the ion motion in the Penning trap using the Brown-Gabrielse invariance theorem:
νc2 = ν+2 + νz2 + ν-2.
The axial frequency νz of the stored 40Ar13+ is directly observed by non-destructive image current detection. The modified
cyclotron frequency ν+ and the magnetron frequency ν- are measured by sideband coupling.
The determination of the Larmor frequency νL is based on the non-destructive detection of spin transitions (spin flips)
using the continuous Stern-Gerlach effect.
The experimentally obtained value of g=0.66364845532(93) is in agreement with the new theoretical prediction
of 0.66364812(58), calculated under participation of the
theory division of Christoph Keitel at MPIK .
The agreement between theory and experiment represents one of the most accurate tests of many-electron QED contributions
in strong fields and paves the way toward an independent determination of the fine-structure constant α.
Please read more in the article ... >
Further information also in the press release of the MPIK
(idw
).
Laser spectroscopic and theoretical investigation of the discontinuity in charge radii at shell closures
According to the shell-model picture, in atomic nuclei each shell can be filled with a certain maximal number of protons or
neutrons. Completely filled shells define the so called "magic" proton and neutron numbers 2, 8, 20, 28, 50, 82 and
additionally 126 in the case of the neutrons.
In the last years, modern precision measurements of nuclear charge radii have revealed new facets of nuclear structure and
dynamics along isotopic chains, e.g. the kink at shell closures, i.e. the considerably different differential increase
of nuclear charge radii per isotope before and after a magic number.
Most of the discussion pertaining to the kink in charge radii was focused on the N=126 and N=28 shell
closures in the lead (Z=82) and calcium (Z=20) region (see our
news of 08.02.16).
From xenon (Z=54) to tellurium (Z=52) a reduction of the N=82 kink can be observed. Thus, the question arises
whether this reduction with decreasing proton number persists in Z=50 tin isotopes like nuclear density functional
theory (DFT) calculations with Skyrme functionals predict or a rather strong kink can be experimentally found as suggested
by Fayans functional predictions for tin (Sn) and the neighboring Cd chain (see our
news of 04.09.18).
In a recent article published in Physical Review Letters C. Gorges et al. present new data on charge radii for the
even-even 108-134Sn isotopes based on high-precision laser spectroscopic measurements, crossing for the first time the
doubly magic N=82 shell closure in the tin isotopic chain.
Collinear laser spectroscopy was performed on the neutron-rich tin isotopes using the atomic transitions
5p2 1S0 -> 5p6s 1P1 ("SP", 452.5 nm) and
5p2 3P0 -> 5p6s 3P1 ("PP", 286.3 nm) at the
COLLAPS
experiment situated at ISOLDE/CERN
,
Geneva.
For the determination of the isotope shift, spectra of the even isotopes 112-134Sn were recorded for both transitions,
while 108,110Sn were only observed in the PP transition. Reference scans of 124Sn were carried out regularly between
the other isotopes.
From the isotope shifts, the changes in mean-square nuclear charge radii of the measured even-A tin isotopes
with respect to the reference isotope 124Sn were extracted. Below 132Sn, the evolution of the nuclear charge radii of
the even tin isotopes was found to be almost linear, with a small curvature. A clear kink occured at doubly magic
132Sn that is indicative of a neutron shell closure. This is similar to what has been observed at N=82 in other isotopic
chains with larger proton numbers - e.g. tellurium - , and at the N=126 shell closure in doubly magic 208Pb.
The experimental results were analyzed by nuclear density functional theory (DFT) using a Skyrme functional (SV-min)
and a recently developed Fayans functional Fy(Δr,HFB) involving gradient terms in surface and pairing energies.
The experimentally found parabolic behavior of the charge radii below the N=82 shell closure is almost absent in SV-min and
over-expressed in Fy(Δr,HFB).
SV-min produces a flat trend for the charge radii along the tin isotopic chain while Fy(Δr,HFB) predicts a kink for
132Sn as well as the kink at 208Pb in accordance with experiment. A multiple correlation analysis leads to the conclusion
that both kinks are related to pairing and surface effects, that are considered in the new Fayans energy density functional.
Please read more in the article
and the press release of TU Darmstadt
(in German).
The article has been selected as "Editor's Suggestion".
Further information also in the Synopsis
of the article.
Special issue of Annalen der Physik on the revision of the international system of units
The properties of our world are determined by the values of the fundamental physical constants, for example,
the fine-structure constant, Planck's constant, the speed of light, and the elementary charge.
The definition of what to consider as a fundamental constant is still debatable and thus it still remains
unanswered how many constants are really fundamental and needed to describe Nature.
It is even not known whether the fundamental constants are really constant or change over time and space.
Therefore, during the last years, for example, a series of high-precision atomic clock experiments have been
performed and put new constraints on the possible time variation of the fine structure constant and
the proton–electron mass ratio.
Following a suggestion of Max Planck in 1900, fundamental constants can be used to define "natural" physical units.
The metre is e.g. defined as the length of the path travelled by light in vacuum in 1/299792458 of a second.
On 16 November 2018, the 26th meeting of the General Conference on Weights and Measures in Versailles
decided to establish the international system of units, the SI, by fixing the numerical values of seven
"defining constants". In particular, the kilogram prototype, a platinum-iridium cylinder, which currently still
defines the kilogram, will be replaced by a fixed value of the Planck constant h.
These changes will come into effect on 20 May 2019, the World Metrology Day. On this occasion "Annalen der Physik" has
just published a special issue "The Revised SI: Fundamental Constants, Basic Physics and Units".
It is edited by our division director Klaus Blaum, Dmitry Budker (Johannes Gutenberg University of Mainz),
Andrey Surzhykov (Technical University and Physikalisch Technische Bundesanstalt – PTB, Braunschweig), and
Joachim H. Ullrich (President of the PTB, the National Metrology Institute of Germany).
The special issue is one of the events of the World Metrology Day 2019 .
It contains invited reviews by world-leading experts who consider the use of fundamental constants
for the definition and realization of the seven base units of the new SI. High-precision experiments
aiming for the determination of the fundamental constants are also in the focus of the special issue.
Please read more in the editorial
and the articles
of the special issue of Annalen der Physik.
Review article on the Alphatrap experiment at MPIK
In a recent article published in The European Physical Journal Special Topics S. Sturm et al. describe the technical architecture and the performance of the Alphatrap experiment at MPIK Heidelberg and summarise the experimental measurement possibilities.
Quantum electrodynamics (QED), the interaction of electrically charged particles via the exchange of photons, served as a blueprint for
the development of the other quantum field theories. Thus, the experimental verification of QED is of major importance and interest.
The Alphatrap experiment at MPIK Heidelberg is a next-generation setup aiming to probe the boundaries of validity of quantum
electrodynamics and the Standard Model of Physics with very high precision under conditions as extreme as possible, especially extremely
high field strengths.
The bound electron in a highly charged ion (HCI) is exposed to the extreme field strengths of the nucleus reaching up to 1016 V/cm in hydrogenlike lead 208Pb81+. The g-factor of the bound electron (the strength of the magnetic interaction of the electron spin) can be predicted very precisely by bound-state QED (BS-QED) and also can be experimentally measured with comparable high accuracy. A comparison of the theoretical prediction of the electron g-factor with a measured value can yield the most stringent test of the Standard Model in strong fields.
A Penning trap is formed by a superposition of an electrostatic quadrupole potential and a homogeneous magnetic field. Advanced Penning
trap systems are used to measure the g-factors of stored charged particles with high precision. Over the past years the predecessor
Penning trap experiment of Alphatrap at the Johannes Gutenberg-University in Mainz, Germany has performed a series of measurements
on different elements and charge states. The g-factor determination in hydrogenlike silicon 28Si13+ has provided the most stringent
test of QED in strong fields to date.
For Alphatrap at MPIK Heidelberg, the design of the Mainz experiment has been upgraded with novel techniques to allow ultra-high
precision experiments with heavy HCI. Alphatrap allows the injection of ions from external ion sources, such as the Heidelberg EBIT,
which can eventually provide HCI up to hydrogenlike lead 208Pb81+.
The g-factor measurements with Alphatrap are based on a double Penning-trap technique. Alphatrap's double Penning-trap system
involves the so called "precision trap" and "analysis trap". The g-factor (magnetic moment) of a stored HCI can be derived from
the frequency ratio νL/νc of its Larmor frequency νL (precession frequency) and cyclotron frequency νc.
The cyclotron frequency νc can be determined from the so-called invariance theorem:
νc2 = ν+2 + νz2 + ν-2.
The axial frequency νz of the stored HCI is directly observed by non-destructive image current detection.
The modified cyclotron frequency ν+ and the magnetron frequency ν- are measured by sideband coupling.
The Larmor frequency νL can be determined by means of spin-transition spectroscopy. The superimposition of an inhomogeneous magnetic
field, called a "magnetic bottle", couples the spin-magnetic moment of the HCI to its axial oscillation frequency and thus allows
to apply non-destructive detection of spin transitions using the continuous Stern-Gerlach effect.
The large magnetic bottle broadens the Larmor resonance and limits the measurement to a relative precision of several
parts-per-million. The double Penning-trap technique solves this problem by using the precision trap (PT) and the analysis
trap (AT). The two specialised traps are interconnected by a transport section. The Alphatrap PT features an exceptionally harmonic
electrostatic potential and a nearly homogenous magnetic field. The PT is used for high-precision measurements of
νc and νL. The analysis trap (AT) with the very strong magnetic inhomogeneity (magnetic bottle) is deployed for spin state
detection of the highly charged ion.
The novel, highly harmonic trap has been commissioned and shows excellent performance. Recently, the first determination of the spin-state of a boronlike 40Ar13+ ion was demonstrated, which paves the way to the first g-factor measurements in Alphatrap.
Alphatrap is a uniquely versatile experiment. Besides its central goal of testing bound-state QED in the strongest fields by comparing
the measured g-factor of heavy highly charged ions with its prediction by theory, Alphatrap enables a whole range of intriguing
measurements.
In 2014, S. Sturm et al. reported on the high-precision determination of the atomic mass of the electron by Penning trap
measurements at Mainz (see our news of 20.02.14). The large Alphatrap precision trap allows to reduce the dominant uncertainty
of this experiment and will give the opportunity to further improve the accuracy of the result of the Mainz experiment.
Furthermore, the new Penning trap setup allows for sympathetic laser cooling of the HCI as well as performing laser spectroscopy
of highly forbidden, narrow transitions, which are difficult or impossible to address in other experiments.
Consequently, the researchers expect, that Alphatrap will perform intriguing experiments in a previously unexplored regime and
shed light on physics in the strongest electromagnetic fields.
Please read more in the article ... >
First measurements of heating rates in a cryogenic Penning trap
Trapped charged particles are well-isolated from environmental influences and thus are ideally suited for versatile
applications in metrology and quantum information processing.
Trapped ions are also highly sensitive probes to test fundamental symmetries and to search for physics beyond the
standard model. For this purpose, the
BASE collaboration
at CERN, which includes members of our division, performs high-precision
experiments with stored charged
particles based on precise frequency measurements using advanced multi-Penning trap systems and novel measurement
techniques. Our experiments make high-precision comparisons of the fundamental properties of protons and antiprotons
and provide stringent tests of CPT invariance (C: Charge, P: Parity, T: Time) in the baryon sector. In 2017, BASE performed
the most precise measurements of the proton magnetic moment (see our news of 24.11.17)
and of the antiproton magnetic moment (see our news of 18.10.17).
These high-precision measurements require low-noise conditions much more
demanding than in any other ion trap experiment. Our precision experiments become possible only in cryogenic ultra-low-noise
Penning traps with energy stabilities corresponding to a parasitic transition rate of maximal two motional quanta over
several minutes of measurement time.
In a recent article published in Physical Review Letters M. J. Borchert et al. report on the characterization of the
electric field fluctuations in a cryogenic Penning trap by explicit measurements of cyclotron quantum transition
rates of a single antiproton using the continuous Stern-Gerlach effect. The measurements were conducted in the cryogenic
spin-state analysis trap of BASE (BASE: Baryon Antibaryon Symmetry Experiment ).
The analysis Penning trap is realized using a superconducting magnet at 1.945 T combined with a quadrupolar electrostatic
potential provided from a set of five carefully designed cylindrical electrodes with an inner diameter of 3.6 mm.
The central ring electrode is made out of a cobalt-iron alloy generating the magnetic inhomogeneity of 272 kTm-1 used for
application of the continuous Stern-Gerlach effect.
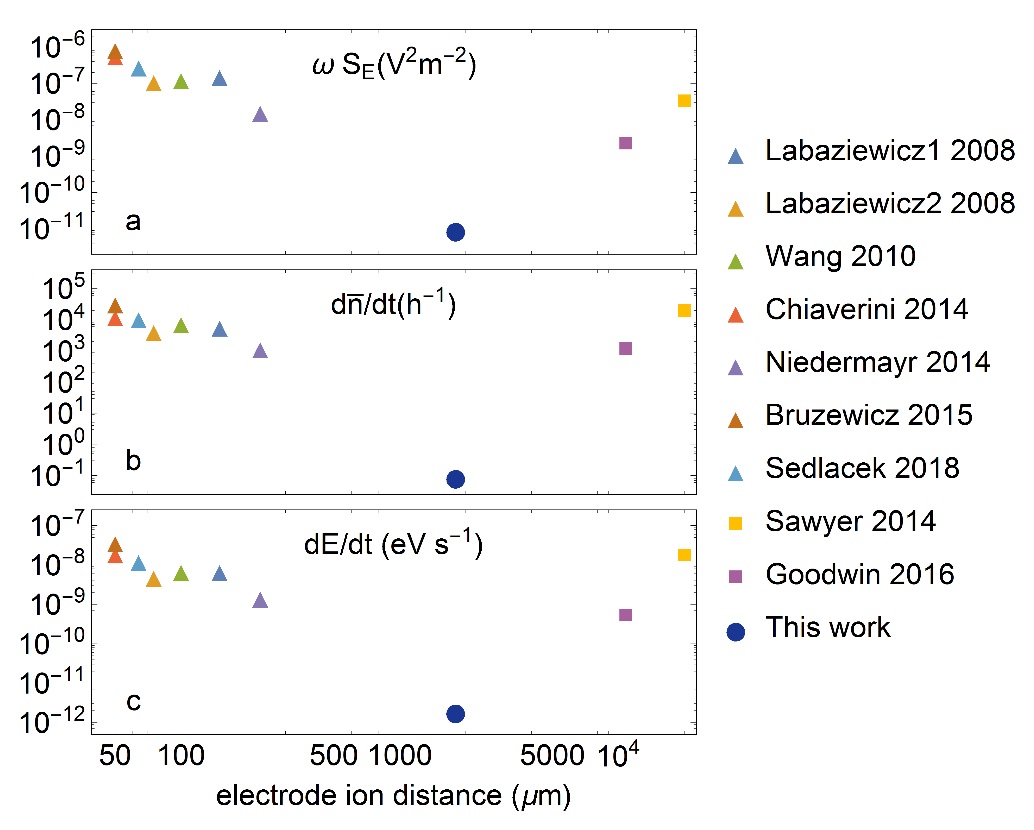
The trajectory of a single antiproton stored in the analysis trap is composed of the modified cyclotron motion at ν+ and
the magnetron motion at ν- perpendicular to the magnetic field, and the particle oscillation along the magnetic field lines
with axial frequency νz. νz was directly observed by non-destructive image current detection applying active electronic
feedback cooling which enabled measurements at low axial temperature and high axial frequency stability.
Due to the continuous Stern-Gerlach effect the antiproton's axial frequency νz becomes a function of the radial quantum
states and transitions in the quantum states lead to axial frequency shifts. Thus, cyclotron quantum transition
rates can be determined by axial frequency measurements.
An axial frequency stability analysis for an antiproton at low radial energy was carried out by evaluating the Allan
deviation σ_νz(τ). At small averaging times (τ < 100 s) the axial frequency fluctuations are dominated by frequency
measurement noise and voltage fluctuations. In contrast, at long averaging times (τ > 250 s), the Allan deviation
is dominated by transition rates in the modified cyclotron mode, which describe a nearly undirected random walk.
An absolute cyclotron transition rate of 6(1) quanta/hour was extracted from the data.
The observed transition rates were related to the spectral density of electric field noise SE(ω+) acting on the
particle's cyclotron motion. An upper limit for SE(ω+) of
7.5(3.4)·10-20 V2m-2Hz-1 was obtained, which is far
below the results reported by cryogenic Paul trap and room temperature Penning-trap experiments.
ω·SE(ω), i.e. the electric-field noise spectral density scaled by angular trap frequency, was determined to be below
8.8(4.0)·10-12 V2m-2, which is by a factor of 1800 lower than the best reported Paul trap heating rates and by a
factor of 230 lower than the best Penning trap heating rates. Furthermore, the determined energy increase dE/dt is on the
order of peV/s. This corresponds to the highest energy stability of a particle in any ion trap experiment.
Based on heating rate measurements at various particle orbits (i.e. magnetron radii ρ-), fluctuations in the trapping
field, caused by residual electrode voltage noise SV, were identified as the dominant heating mechanism.
"Anomalous heating", an effect limiting the scalability of quantum information experiments,
was not observed within the measurement resolution. Parasitic coupling of stray EMI (Electromagnetic
Interference) noise onto the trap electrodes is presumably the dominant source of SV and thus electric field
fluctuations in the analysis trap. The researchers intend to enhance the sensitiviy of future Penning trap measurements
by achieving even lower heating rates.
Please read more in the article ... >
Further information also in the press releases of BASE
and the Trapped-Ion Quantum Engineering group
.