Research: Low-Level Physics
Very Low Activities of natural and man-made Radioisotopes
Low-Level Techniques are experimental techniques which allow to investigate very low
activities of natural, man-made, and cosmogenic radioisotopes, e.g.:
- Material screening (Ge spectroscopy, ICP-MS, NA)
- Surface screening (alpha, beta, gamma spectroscopy)
- Study of (radioactive) noble gases (diffusion, emanation)
- Purification techniques (liquids, gases)
- Background event rejection techniques (alpha/beta/gamma/neutron discrimination, PSA)
- Background modeling in experiments (MC simulations)
Low-level techniques are naturally coupled with the experiments looking for rare events (detection
of neutrinos, search for dark matter, search for neutrino-less double beta decay, search for proton decay, ...),
where the backgrounds identification and reduction plays a key role. At the Max Planck Institute for Nuclear Physics (MPIK) there is a long tradition and a lot of expertise in that field. Several extremely sensitive techniques have been developed in the course
of the GALLEX/GNO, BOREXINO,
Heidelberg-Moscow, LENS and
GERDA experiments.
Low-Level Laboratory
The Low-Level Laboratory of the MPIK offers ideal conditions for detector
development of low-background experiments. The combination of nucleonic component reduction and active cosmic veto
shielding enables e.g. in the Ge-spectroscopy a strong background reduction. Here the development of several experimental
methods for the solar neutrino experiments GALLEX/GNO, BOREXINO, LENS and for the Heidelberg-Moscow double beta decay
experiment has been started.
The laboratory was constructed in 1967 and completely renovated in 2007. It is equipped e.g.
with special heavy-load shelves for underground storage of high purity materials (in order to reduce cosmic activation),
a heavy-load crane, a central gases supply system, "clean" power (for the detectors operated there), an air conditioning etc.
The laboratory offers a working space of about 100 m2 and it is easily accessible from the basement of the main building via
a 20 m long floor. It has an overburden of 7 m of soil and 0.9 m of concrete corresponding to a depth of 15 meters pf water eqivalent.
The 222Rn concentration of the laboratory air varies between 30 and 70 Bq/m3.
Figure 1: A cross-section of the Low-Level lab at MPI-K in Heidelberg
Figure 2:The expected reduction of the cosmic rays
(and cosmic ray induced background) at different depths with a mark for 15 m w.e.
Ge-spectroscopy
Ge-spectroscopy is one of the most powerful techniques to identify gamma emitters (U and Th chain, 40K, 137Cs, 60Co, ...) and therefore is a perfect tool for material screening. This is due to the excellent energy
resolution (ca. 2 keV) and the very low intrinsic background (high purity crystals). However in order to reach a high detection sensitivity it is
necessary to reduce backgrounds originating from construction materials and from external sources (active/passive shielding, underground locations of
the detectors), to assure reasonably large sample volumes and to provide a precise determination of detection efficiencies
through calibrations and simulations.
MPIK operates several detectors at different locations. The world-wide most sensitive of these are GeMPI-type spectrometers [1] located at the Gran Sasso laboratory in Italy. Due to the extremely low background und the` large
capacity, for high density materials it is possible to reach the detection limits at the level of 10 μBq/kg (U and Th).
Some detectors (called Dario, Bruno, Corrado) are operated in the MPIK underground laboratory [2], however because of the shallow
depth (see above) the lowest measurable concentrations are in the range of 1 mBq/kg.
Figure 3: A cross-section of the GeMPI-1 detector installed in the Gran Sasso underground laboratory (3500 m w.e.). The sample chamber volume is 15 l which in connection with an extremely low background level allows
to investigate concentrations as low as 10 μBq/kg (high density materials). The air lock prevents 222Rn to enter the sample chamber. The crystal has a mass of 2.2 kg and 102 % relative efficiency. The detector was built in cooperation with the Canberra company using only previously selected materials of low radioactivity.
Figure 4: Detectors Dario (left) and Bruno (right) with detection limits in the range of 1 mBq/kg.
Figure 5: Detector Corrado under construction. The copper chamber and the lead shield are visible.
222Rn measurements
222Rn is one of the most dangerous isotopes for many low-background experiments. It belongs
to the 238U chain and as an inert noble gas it has typically high mobility (diffusion coefficient) allowing easy escape
from bulk materials and diffusion into active parts of detectors. Together with its short-living daughters 222Rn generates
radiations within a wide energy range. At the end of the decay chain high activities of the longer living daughter 210Pb (T1/2= 22.3 y) may accumulate, which forms a subchain with e.g. the very volatile daughter nuclide 210Po.
The activity of 222Rn can be measured using for example low-background proportional counters designed
originally for the GALLEX/GNO experiment [3]. They are hand-made out of very pure quartz and have an active volume of about 1 cm3.
The background and detection efficiency (for alpha particles) for energies above a 50-keV threshold is 0.1 - 1 count per day
(depending on the individual counter) and 50 %, respectively. Counters are filled with radon samples using a special
gas filling line. 222Rn emanation can be studied using special emanation chambers. Two stainless steel electro-polished and
completely metal-sealed vessels with volumes of 20 and 80 l are available [4]. For samples up to 1 l volume small glass vials
could be used. The absolute detection limit obtained for emanation tests is at the level of 100 μBq (∼50 atoms).
To measure the radon concentration in gases a system called MoREx (Mobile Radon Extraction Unit) has been constructed [5]. It uses the advantage of the 222Rn pre-concentration in charcoal traps at low temperatures. In connection with low-background counting
it is possible to analyze gas contaminations down to ∼0.5 μBq/m3 (STP).
To analyze 222Rn and 226Ra in water STRAW (System for the 222Rn and 226Ra
Assay of Water) can be used [6]. Its main component is a 450-l tank where typically 300 l of water is degassed from 222Rn using
radon-free nitrogen. The 222Rn is collected in a charcoal trap, which is then connected to line for sample
processing and counter filling. The achievable sensitivities are at the level of 0.1 and 0.8 mBq/m3 for radon and radium,
respectively (for 222Rn measurement the detection limit is better due to a short extraction process when the blank value of the
vessel - ∼0.8 mBq - can be neglected).
For high sensitivity online 222Rn monitoring it is possible to use large volume electrostatic chambers [7].
Here the detection principle is based on the fact that most of the radon daughters just after creation are positively charged and
in a strong electric field can be collected on a surface of a PIN diode, which registers their alpha decays. The detection limits
of the two chambers operated by MPI-K are at the level of 1 mBq/m3 and ∼0.1 mBq/m3, respectively. The first detector
is located in Hall C of the Gran Sasso laboratory and monitors the radon concentration in the BOREXINO nitrogen blanket. The other one
with improved sensitivity is currently testing the radon concentration in the argon blanket of the GERDA tank.
Very convenient are also scintillator chambers (Lucas cells) [8], especially for 222Rn monitoring in the
underground laboratories, clean rooms etc. They less sensitive due to the scintillator impurities (typically ZnS(Ag)is used containing 226Ra). Achievable sensitivity reaches 0.5
Bq/m3.
Figure 6: One of the GALLEX/GNO HD-II proportional counters used for 222Rn measurements. The sample with a counting
gas (P10) is pushed inside the active volume through a capillary tube using mercury.
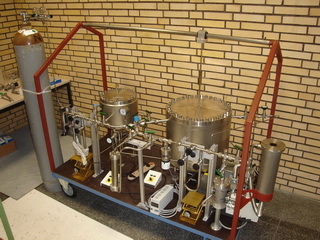
Figure 7: A system of two emanation chambers: It is equipped wit a pumping system (pre-vacuum and turbo pump),
a He purification trap and a trap used to collect extracted radon.
Noble gas mass spectrometry
Other noble gases, which can be considered as sources of background e.g. in BOREXINO are for example 39Ar or 85Kr present in nitrogen as traces. In principle their concentrations could be measured as that
for radon, using low-background proportional counters, however the pre-concentration does not work for them efficiently
enough, and achievable detection limits would be here much poorer. Another solution is to look for natural Ar and Kr
using a highly sensitive noble gas mass spectrometer. Concentrations of 39Ar and 85Kr can be later reconstructed
taking into account their fairly well known specific activities. Ar/Kr is detected using a VG-3600 mass spectrometer devoted
to investigate rare gases at low concentrations in terrestrial and extraterrestrial samples. Besides the mass spectrometer,
the system consists of a sample preparation and a sample purification section. In the first one the volume of the
investigated sample can be determined (or if necessary divided into smaller fractions), in the second one the sample
is purified from reactive gases before it is introduced into the spectrometer. For final purification Al/Ti getter
pumps are used. The calibration is performed with air or Ar/Kr standards. Taking into account the intrinsic background
of the whole system and measuring 1 cm3 of nitrogen the following detection limits coud be achieved: 10-9
m3/m3 for Ar in N2 (∼1.4 nBq/m3 for 39Ar in N2) and 10-13
m3/m3 for Kr in N2 (∼0.1 μBq/m3 for 85Kr in N2) [9].
Some applications in BOREXINO
226Ra in the Inner Vessel foil: The purity requirements for the construction materials in
BOREXINO, especially for the scintillator and its nylon vessel were very severe. The allowed contamination for the second one with
238U was at the level of 1 ppt, corresponding to ∼12 μBq/kg for 226Ra (in secular equilibrium). This limit
could not be reached with any Ge-spectrometer (the foil is a low density material) and a new technique had to be developed. It is based on
the combination of the 222Rn diffusion and emanation measurements and uses the fact that the radon diffusion coefficient in nylon
depends very strongly on its water content. Measuring emanation rates for dry and wet material one could determine the surface and bulk
222Ra concentrations at the levels of 0.5 μBq/m2 and 10 μBq/kg, respectively [10]. In the frame of those
investigations a proper material for the vessel was identified. It was also shown that 238U and 226Ra in nylon were
in disequilibrium.
Nitrogen: One of the most important applications of ultra-pure nitrogen in BOREXINO was the
scintillator purification. The required purity was: 7 μBq/m3 for 222Rn,
0.4 ppm and 0.2 ppt for Ar and Kr, respectively. A measuring campaign also taking into account the transportation logistic identified the company SOL to deliver the proper quality [11]. Beside the mentioned ultra-pure nitrogen BOREXINO uses also 222Rn-free- and boil-off nitrogen (obtained from technical quality LN2) produced with the rate up to 100 m3/h [5].
Some applications in GERDA
Ge-spectroscopy of steel samples: As in many other experiments germanium spectroscopy was applied in GERDA to
screen construction materials like steel (for the cryostat) or copper (for detector holders and shields). The screening was
done in Heidelberg and in Gran Sasso using the GeMPI detectors. One of the most important achievements was the finding of steel
with surprisingly low U and Th contamination of the order of < 0.1 - 1 mBq/kg [12]. Batches with the lowest concentrations
(< 0.1 - < 0.4 mBq/kg) were used to construct the cylindrical part of the cryostat, the others for the top and bottom heads.
Low U and Th content in steel allowed thickness reduction of the copper shield installed inside the vessel (keeping the same
background index).
222Rn measurements:
Many samples like gaskets of different kinds, valves, oxygen/water adsorbers or steel welds were investigated. For some of them, like
e.g. welds also different cleaning procedures were tested (etching, electropolishing). Since LAr will be used as a cooling medium and
a passive shield for the Ge-detectors a big effort was also put into measurements of 222Rn content in argon of different
qualities and into tests of argon purification from radon. In general it has been found out that the concentrations of 222Rn
in argon are in the mBq/3 range, almost two orders of magnitude more than in nitrogen. The dependency on the gas
quality (grade) is very weak. Most likely, LAr is stored and distributed after production in different containers before it reaches the consumer.
One of the most important background components in the experiment is 222Rn emanation
of the cryostat. According to the simulations it should not exceed 14 mBq (taking into account segmentation of the diodes, which helps to
reject some background events). The first test has been performed already at the site of the company, who built the vessel. The result was
∼30 mBq. After additional cleaning (etching) that value was reduced to the acceptable level of 13.5 mBq. The next measurements
will be done after internal Cu shield mounting in Hall A at Gran Sasso.
References
[1] Heusser G. Laubenstein M and Neder H 2006 Radioactivity in the Environment, edited by P. P. Povinec and J. A. Sanchez-Cabeza, Elsevier, Amsterdam 495-510
[2] Heusser G. 1995 Ann. Rev. Nucl. Part. Sci. 45: 543-590
[3] Wink R. et al. 1993 Nucl. Instr. Meth. A 329 541-541
[4] Rau W. and Heusser G 2000 Appl. Rad. Isot. 53 371375
[5] Heusser G. et al. 2000 Appl. Rad. Isot. 52 691
[6] Simgen H. et al. 2003 Nucl. Instr. Meth. A 497 407
[7] Kiko J. 2001 Nucl. Instr. Meth. A 460 272
[8] Wojcik M. Wlazło W. 1994 Nucl. Instr. Meth. A 345 351
[9] Zuzel G. et al. 2004 Appl. Rad. Isot. 61 197-201
[10] Zuzel G. et al. 2003 Nucl. Instr. Meth. A 498 240
[11] Simgen H. and Zuzel G 2007 AIP Conf. Proc. 897 45
[12] Maneschg W. et al. 2008 Nucl. Instr. Meth. A 593 448-453
Contact:
- Dr. Wolfgang Hampel
Tel: +49 6221 516464
E-Mail: wolfgang.hampel [at] mpi-hd.mpg.de
- Dr. Gerd Heusser
Tel: +49 6221 516464
E-Mail: gerd.heusser [at] mpi-hd.mpg.de
-
Dr. Hardy Simgen
Tel: +49 6221 516530
E-Mail: Hardy.Simgen [at] mpi-hd.mpg.de