Research: Theoretical Neutrino Physics
The Potential of Future Neutrino Experiments
Existing technology will make it possible to perform more precise
measurements of neutrino masses and mixing parameters in the future.
However, neutrino physics is still a rather dynamic field where
new ideas for improved experiments keep emerging and new windows of research open up.
The group has been at the forefront of some of these ideas – in addition to
further developing them and evaluating the physics potential
of various options. Recent examples are investigating the new physics potential of elastic coherent neutrino-nucleus scattering, which ha been observed for the first time in 2017, the speculative proposal to use the Mößbauer effect for neutrino oscillation measurements, the possibility of
using pure electron (anti-)neutrino beams from beta-decaying isotopes
or to use two identical detectors in reactor experiments in
order to significantly reduce systematic errors.
Fig. 1: Possible effects of Non-Standard Interactions
(NSIs) in future neutrino oscillation experiments: Shown
are the values of the parameter sin2 (2θ13)
extracted from two different future experiments. Two assumed true values are
marked by the stars. NSIs can be responsible for a common offset
(indicated by the arrow along the diagonal axis) or even inconsistencies
(indicated by the arrow orthogonal to the diagonal).
Different ideas for future neutrino experiments have often
been discussed individually and at various levels of detail.
However, the large scale of these experiments means that, to obtain the
best results, it is necessary to evaluate their physics potential in
realistic simulations and also to compare them on an equal footing.
The software package
GLobES (Global Long baseline Event
Simulator) was developed by the group for this purpose.
This software is used and further refined by the group, but it is
now also widely used by others. GLobES is based on an abstract experiment
definition language which allows the inclusion of all relevant
aspects of existing and future experiments. It performs an event
rate based evaluation of the physics potential of the experiment
under consideration. Various simulations of reactor experiments,
beam experiments, sensitivities to non-standard interactions
(NSIs, see
Figure 1), as well as synergetic combinations of different
neutrino sources have been performed. A prominent example
is the combination of new long-baseline experiments with new
reactor experiments, which in a few years could even lead to the
first measurement of leptonic CP violation. These simulations not
only indicate the best experimental strategies, but they also show
how the situation can change depending on the progress
in detector and source development. The simulations are therefore
not only important for the planning of projects, but also for
source and detector R&D. The simulations of the International
Neutrino Factory Scoping Study (ISS) and of the
Neutrino Factory International Design Study (IDS) are also mostly
based on GLobES.
In parallel with these numerical tools, analytic
approximations have also been developed by the group in order to
systematically understand and explore the multi-dimensional parameter
space of three flavour oscillations in matter. In addition, other
simulations were performed which showed the potential to
determine the overall mass scale of
neutrinos by neutrinoless double beta decay when combined
with cosmological limits.
Neutrino Physics and the Origin of Flavour
Over roughly the last decade, a consistent picture of neutrino
oscillations has emerged and the discovery of surprisingly
large mixings has shed new light on the flavour problem. However, the
origin of flavour is still far from being understood, even
though apparent regularities in fermion masses (see Figure 2)
and mixings suggest that eventually three generations, neutrino masses
and mixings, charged lepton masses as well as quark masses and
mixings will be explained by some theory of flavour.
The structure and properties of the see-saw mechanism(s) can be
constrained by the particular structure of lepton mixing.
In the future,
precision measurements of neutrino parameters will provide
further very valuable information. By combining measurements of
quark masses and mixings with the precisely determined leptonic
parameters, there will be the strong potential to discriminate
theories which unify the generations based on flavour symmetries.
In addition, there are also strong indications pointing to the
unification of the gauge couplings at high energies in enlarged
versions of the Standard Model, such as the Minimal Supersymmetric
Standard Model (MSSM).
This is a strong hint for some Grand Unified Theory (GUT),
where the known quark and lepton
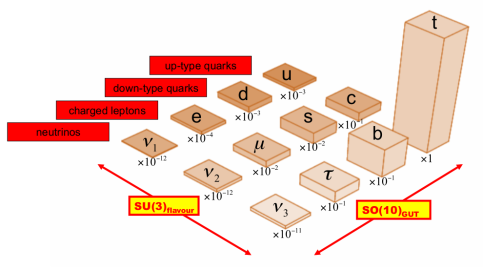
Fig. 2: The fermion content of the Standard
Model: shown are the three generations of the four
types of fermions and their individual masses in
relation to each other. It is also indicated in which
direction GUTs (here SO(10)) and flavour symmetries (here SU(3)) work.
fields of the same generation are unified into GUT multiplets. Information on neutrino masses is very useful here, since right-handed or other sterile neutrino fields require different GUT groups and/or GUT representations. Due to the indications for a GUT and flavour unification the group has studied theories which embed into a GUT or a flavour symmetry or both. High-energy predictions of neutrino mass models are subject to quantum corrections which can in turn lead to significant modifications of the low-energy predictions. These renormalization group corrections of neutrino masses and mixings have been systematically studied for various cases. This will lead to a situation where models of neutrino mass have to cope simultaneously with a number of constraints and a number of very well measured quantities. In addition, such flavour models will be further constrained in the coming years by new results coming from searches for lepton flavour violation, from the LHC and from astroparticle physics. This interplay between theory and experiment may lead to a situation where none or only very few candidate models of flavour survive pushing us closer towards an understanding of flavour. The differences of neutrino mass compared to the other fermion masses in the Standard Model motivate studies of alternative mass generation mechanisms. An approach followed actively by the group is to apply conformal symmetry (or classical scale invariance), in which mass terms, in particular quadratic terms in Higgs potentials, are forbidden at tree-level. Loop-corrections to the potential generate a non-vanishing vacuum expectation value of the scalar fields and thereby generate mass scales.
Non-equilibrium QFT approach to Leptogenesis
An important topic within astroparticle physics is the explanation of the matter-antimatter asymmetry (baryon asymmetry) of the Universe, which can only be produced if the so-called Sakharov conditions are fulfilled. Although it fulfills all Sakharov conditions, the Standard Model of particle physics predicts a baryon asymmetry that is many orders of magnitude smaller than observed. Various extensions of the Standard Model allow for the generation of a baryon asymmetry of the correct magnitude. The most convincing mechanism is leptogenesis which is related to the see-saw mechanism which explains the small neutrino masses. Within this scenario a lepton asymmetry is produced initially. Subsequently it is converted into to the desired baryon asymmetry. In the past, the division has contributed several interesting realizations of the leptogenesis scenario and studied the interplay of leptogenesis and neutrino mass models. The most popular realization is based on the decay of heavy Majorana neutrinos whose distribution function deviates from thermal equilibrium due to the rapid expansion of the Universe. The products in this decay are a lepton and a Higgs particle which known from the Standard Model. Recent activity was inspired by the fact that it is unclear if the extreme conditions of the early Universe, at the time of leptogenesis, permit the use of thermodynamics and Boltzmann equations for the computation of the baryon asymmetry.
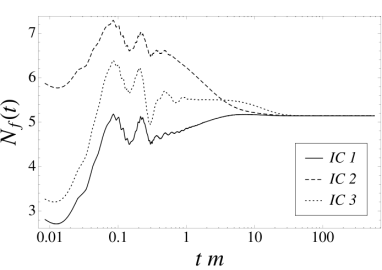
Fig. 3: In-medium contributions to the heavy Majorana neutrino decay amplitude.
The range of applicability of this traditional approach may be determined using methods of non-equilibrium quantum field theory (NEQFT). These allow one to calculate corrections to the results of the conventional analysis and unveil a number of qualitatively new effects. In contrast to the traditional approach, which operates with distribution functions of on-shell particles, NEQFT deals with two-point correlation functions - spectral functions and statistical propagators - of quantum fields. In the language of the conventional approach the former determine the on-shell condition, whereas the latter carry information about the particle distribution functions. In general this is an oversimplification: the two-point correlation functions are not restricted to the mass-shell and contain in general more information. Therefore, they are well suited for the analysis of one of the main ingredients of leptogenesis - the CP-violating mixing of the heavy neutrinos. This is particularly important for the calculation of the CP-violation in the popular scenario of resonant leptogenesis. Furthermore, the two-point correlators automatically account for medium effects and represent an excellent starting point for the computation of the effective in-medium CP-violating parameters, masses and decay widths. Yet another advantage of the NEQFT formalism is the self-consistent structure of the corresponding dynamical equations - Kadanoff-Baym equations - which enables us to study interesting non-equilibrium effects inaccessible in the conventional approach.
All in all, the NEQFT formalism represents a powerful tool which allows us to reach far beyond the state of the art in the analysis of the matter-antimatter asymmetry generation. In particular, it has been shown for a simple toy model of leptogenesis that the NEQFT approach is free of the double-counting problem, which in the canonical approach leads to a generation of nonzero asymmetry even in complete thermal equilibrium. In a hot and dense medium of the early Universe masses, decay widths and CP-violating parameters of the heavy neutrinos receive thermal corrections. Another qualitatively important result is that dependence of these quantities on the distribution functions of the Higgs and leptons differs from the one obtained earlier in the framework of Equilibrium Quantum Field Theory. This also leads to a quantitatively different result for the CP-violating parameter and hence the generated lepton asymmetry. The corrections are particularly large in the case of quasi-degenerate mass spectrum. For instance at temperatures of order of the mass of the heavy decaying particle they may reach several 100%. We are currently working on the generalization of the obtained results to the physically interesting case of the Standard Model supplemented by three heavy Majorana neutrinos.
Supernova Neutrinos
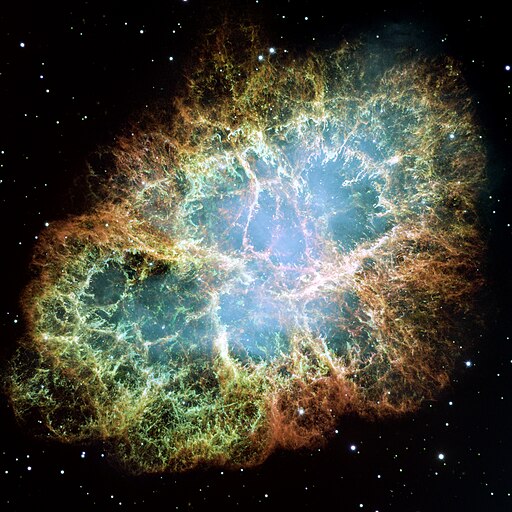
Fig. 4: False color image of a supernova remnant namely the crab nebula.
A star that explodes as a supernova emits 99% of its energy as neutrinos. Still, the remaining energy is enough to outshine an entire galaxy. In the case of a galactic supernova, it will give rise to thousands of events in neutrino detectors on Earth and give us a unique opportunity to learn about supernovae as well as neutrinos. In the division, we are investigating what can be learnt from supernova neutrinos, and we are also working on some of the challenging physics that arises when neutrinos propagate at such high densities.
We only expect to see a few galactic supernovae every century, and since we have no means for predicting when the next galactic supernova will happen, we need to be prepared to get the most of it when it occurs. One way to do this is to use neutrinos as a trigger for optical and gravitational searches. The neutrinos arrive hours or days before the light because they travel freely through the outer parts of the star. By using the precise timing of neutrino detectors at different locations, the supernova can be located on the sky through triangulation.
The precise timing of a supernova neutrino signal can also be used to determine the neutrino mass. By comparing the travel time of neutrinos and gravitational waves or neutrinos of different energy, the delay induced by a finite neutrino mass can be identified. This gives either a measurement of the neutrino mass or an upper bound.
Supernovae can also be used as a powerful laboratory to test beyond Standard Model physics due to the extreme densities and high energies. The presence of a sterile neutrino with keV mass would influence the supernova dynamics and could lead to spectacular new signals from supernovae.
Furthermore, the large number of neutrinos that is emitted by the supernova provides a very unique environment to study how neutrino flavours change. By describing neutrinos using wave packets, the effects of decoherence on supernova neutrinos can be studied. The large number of neutrinos may give rise to what is known as collective oscillations, where neutrino flavour conversion can occur very rapidly. Whether collective oscillations are present in supernovae is still an open question, which is approached with numerical simulations, analytical models and linear stability analysis.
Neutrinos as probes of new physics
Standard Model extensions which describe neutrino masses and mixing have very interesting indirect connections to baryogenesis, lepton flavour violation, lepton number violation, B+L violation, sterile neutrinos, left-right and/or supersymmetric extensions of the Standard Model, electro-weak symmetry breaking, Grand Unified Theories, extra dimensions, Dark Matter and Dark Energy. Neutrinos are also a direct and unique probe into the early Universe and also into supernova explosions, the burning of stars, reactor physics and geophysics. The whole spectrum of such topics has been, and will continue to be studied, and it is expected that through the combination of theoretical developments and experimental results the group will be able to contribute in a broad way to our understanding of the Universe and physics beyond the Standard Model.