Astrophysics with H.E.S.S.
The basic physics goal of the H.E.S.S. experiment is to explore the production and propagation of high-energy particles in the Universe—i.e. to explore the Non-thermal Universe.
The Non-thermal Universe
Much of the radiation propagating in the Cosmos, and incident on the Earth, is thermal radiation generated in hot objects such as stars. Under extreme conditions, thermal radiation can reach into the keV energy range and beyond. It is well-known, however, that certain particle populations in the Cosmos cannot result from thermal processes, and must instead be produced by collective mechanisms, focusing the energy outflow from a source onto a relatively small number of particles. The best-known example of a non-thermal particle population are the cosmic rays. Their power-law spectrum shows no indication of a characteristic (temperature) scale, and their energies —up to 1020 eV and above—are well beyond the capabilities of any conceivable thermal emission mechanism.
Much of the Non-thermal Universe remains to be explored, and while the sources and collective acceleration mechanisms for particles of TeV energies and beyond are subject of much speculation and theoretical work, the experimental identification of sources and of acceleration mechanisms remains challenging. Before the advent of advanced instruments such as H.E.S.S., only few—and possible atypical—objects had been detected, and even less had been studied in any detail.
At the same time, however, the Non-thermal Universe is of significant importance for our understanding of the Universe, its objects, and their evolution. In our Galaxy, e.g., the energy density of cosmic rays is comparable to the energy density of starlight, of interstellar magnetic fields, and of the kinetic energy density of interstellar gas. In interplay between cosmic rays and magnetic fields influences the evolution of galaxies. Nonlinear amplification mechanisms transform a significant fraction of the kinetic energy released in supernova explosions into energies of highly relativistic particles.
The primary goal of the H.E.S.S. experiment was and is to provide the experimental basis for an improved understanding to the acceleration, propagation and interactions of such non-thermal populations of particles.
Using high-energy gamma-rays to locate cosmic accelerators
Cosmic particle accelerators are believed to accelerate primarily charged particles, such as electrons and ions, by acting on these particles with electric fields or magnetic fields. Acceleration can be a one-shot process, where particles are accelerated in huge electric fields generated, e.g., by rotating neutron stars. Other modes of acceleration result in a slow, but continuous increase in particle energy. In shock waves generated by supernova explosions, e.g., particles bounce between magnetic fields, gaining little bits of energy, and take 100s to 1000s of years until they escape with high energy from the acceleration zone.
High-energy gamma rays are almost always secondary products of the cosmic accelerators. Gamma rays are produced, e.g., when a proton accelerated in the supernova blast wave interacts with nuclei of the ambient medium, generating new particles in the collision, among them π0-mesons, which decay into two gamma rays. If the primary accelerator generates a beam of high-energy electrons, these electrons may undergo bremsstrahlung in the ambient medium, may suffer synchrotron radiation losses in local magnetic fields, or may, via the inverse Compton scattering process, transfer a significant part of their energy to an ambient photon, which then emerges as a high-energy gamma ray.
Compared to the charged particles, which are the primary products of cosmic accelerators, gamma-rays have the substantial advantage that they propagate on straight lines through the universe. The charged particles are deflected by galactic and intergalactic magnetic fields. Gamma-rays detected on Earth therefore point back towards their sources and can be used to locate and study the sources. With charged particles, deflections are so large that over most of the energy regime, the pointing information is completely lost; only at the very highest energies, in the domain of the AUGER experiment, can the directional information possibly be exploited.
The flux of gamma rays from a source region is governed by the density of their charged parent particles, multiplied by the density of the target used to generate gamma rays—the ambient medium, the energy density in magnetic fields, or the energy density in low-energy target photons for the inverse Compton process. The energy spectrum of gamma rays is closely related to the spectrum of the parent particles.
A nice example of how photons can be used to trace high-energy cosmic rays and their sources is provided by the Fermi sky surveys. There, the Milky Way shows up as a continuous band of gamma rays, generated in interactions of the cosmic rays pervading the Milky Way with its interstellar gas. Superimposed on the continuum are point sources, reflecting acceleration sites or unusual concentrations of target interstellar matter. Except for very strong sources, space instruments such as Fermi run out of gamma rays at energies between a few GeV to a few 10s of GeV, due to the limited detection area. This is where ground-based instruments such as H.E.S.S. take over, exploring the gamma ray sky in the very high energy (VHE) domain. With the new H.E.S.S. II telescope with its larger dish and lower energy threshold, a seamless connection to the Fermi range will be possible.
Sources of high-energy particles in the Cosmos
A large variety of sources has been proposed to feed non-thermal particle populations in the universe. Many of them belong to the most extreme spots in the universe, regions where energy densities are huge and where the laws of physics are probed under unprecedented conditions. H.E.S.S. has already detected a large number of new sources - in fact, the majority of all known sources - see side panel. The images below show the sky with the H.E.S.S-detected gamma ray sources, as provided by TeVCat (as of July 2012), and the H.E.S.S. Galactic Plane Survey revealing a large number of Galactic sources. More information is given in the H.E.S.S. Source of the Month, the H.E.S.S. publications, and the H.E.S.S. source catalog.
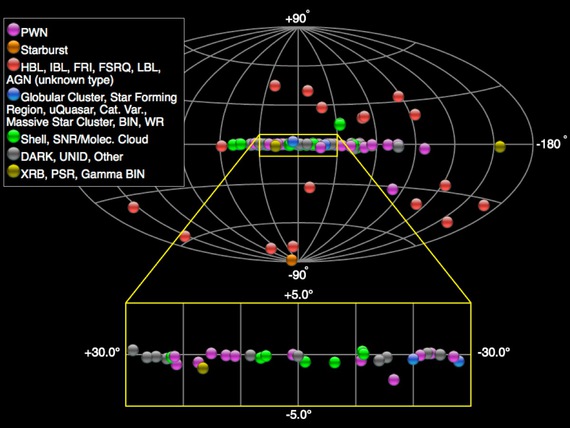
Goal of instruments such as H.E.S.S. is to detect a sufficient number of sources of each type to allow a meaningful taxonomy of sources and a classification of the acceleration mechanisms. For this purpose, the instrument emphasizes the ability to spatially resolve extended sources—most of the source types discussed below are extended objects—as well as good spectral resolution.
Demonstrated or suspected sites of particle acceleration and particle interaction in the Cosmos include:
-- Supernovae
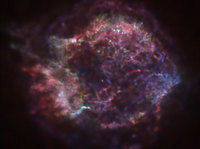
-- Pulsars and pulsar nebulae
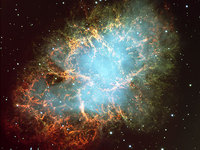
-- Binary stars
Binary systems of stars, where one object accretes matter from the other, may serve as smaller-scale models for active galactic nuclei with the accretion disk surrounding the central massive black hole. In binary systems, the accreting objects could either be a neutron star, or a black hole of few solar masses. Galactic binary systems share many of their characteristics with their big brothers, and are expected to emit high-energy gamma rays, their lower luminosity being compensated by the much smaller distance from the Earth. H.E.S.S. has detected variable emission from the binaries PSR B1259-63 and LS 5039, and has discovered the new gamma ray binary HESS J0632+057.-- Giant molecular clouds
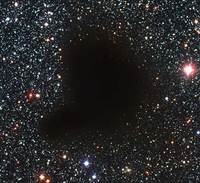
-- Star clusters
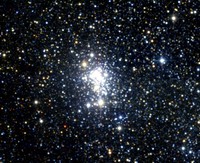
-- "Dark" sources
For some sources of very high energy gamma rays, such as HESS J1507-622, no plausible counterpart at other wavelenghts is known. This may only partly be explained by insufficient exposure by radio and X-ray instruments; potentially these objects represent a new type of source - some authors speculate e.g. that some could be GRB remnants. On the other hand, experience has shown that many of the past unidentified sources have meanwhile found conventional explanations, see e.g. HESS J1813-178 or HESS J1857+026.-- Starburst galaxies
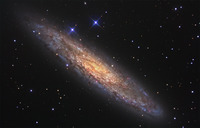
-- Black holes in the centers of active galaxies
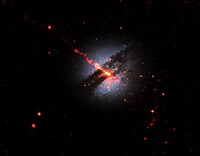
-- Clusters of galaxies
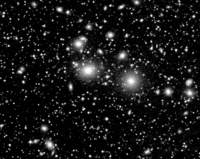
-- Extra-galactic pair halos
If the gamma-ray emission from powerful active galactic nuclei stems to a significant extent from photons with energies > 10 TeV, then these gamma-rays will be absorbed in interactions with diffuse extra-galactic photons. The resulting electron-position pairs are deflected and isotropized in magnetic fields, and may interact with other background photons, scattering these to high energies, ultimately resulting in a cascade process leading to a halo of pairs around the source. The spectrum and spatial extent of high-energy photons emerging from this halo yields information both about the source distance and the photon fields near the source. So far, no pair halo has been detected in VHE gamma rays.Beyond probing cosmic particle accelerators, very high energy gamma ray astronomy addresses a range of topics from astrophysics, cosmology and fundamental physics, such as:
-- Measuring the extragalactic background light
Over extragalactic distances, gamma rays suffer absorption by interactions with infrared and visible extragalactic background light (EBL), resulting in electron-positron pair creation. Since gamma rays of a given energy preferentially interact with photons of a reciprocal energy, the spectrum of EBL photons is imprinted on the spectrum of gamma rays arriving on Earth. The EBL represents the light emitted from all stars since the beginning of the Universe and its level reflects the star formation history in the Universe. However, due to the strong foreground light from within the solar system and the from the Galaxy, the level of EBL is difficult to measure directly. Gamma ray spectra from AGN offer a means to probe this cosmologically interesting quantity, see discussion of the blazars 1ES 1101-232 or 1ES 0229+200.-- The spectrum of cosmic-ray electrons
Contrary to nucleonic cosmic rays, which propagate long distances through the Galaxy, cosmic ray electrons rapidly lose their energy; their sources should be relatively nearby and specific sources such as pulsar wind nebulae may produce specific spectral features such as bumps in the spectrum. The good particle identification capability of Cherenkov telescopes allowed H.E.S.S. to extract the electron yield among the cosmic ray background, for the first time revealing a break or cutoff in the electron spectrum around one TeV.-- Probing fundamental physics with very high energy gamma rays
Lorentz invariance states that the speed of light is identical in every reference frame, and for all photon energies. However, models of quantum gravity predict violations of Lorentz invariance. In quantum gravity, space-time aquires a structure on length scales of the Planck scale (10-35 m), sometime referred to as a space-time foam. Directly probing these scales requires energies of the Planck energy scale, about 1028 eV, far beyond all energies of particles produced in accelerators or observed in cosmic rays. However, even at much lower energies, such a hypothetical space-time foam may influence propagation of particles such as photons, modifying their speed of propagation. Particles will be more susceptible to effects of a space-time foam the shorter their wavelength is, and hence the higher their energy is. For example, studies of the energy dependence of flares in PKS 3155-304 show constant speed of light across the TeV energy range and provide constraints on Lorentz-violating mass scales, approaching the Planck scale.-- Search for dark matter particles and other relics of the Big Bang
An entirely different source of gamma-rays could be various types of heavy relics from the Big Bang, ranging from supersymmetric dark matter particles to monopoles or cosmic strings. Such particles are predicted to have been generated in the Big Bang, and depending on their characteristics, some of them might have survived until the current époque. Decays or - in case of dark matter particles - pair annihilation of such objects would generate a steady flow of gamma-rays, with distinct spectral signatures. H.E.S.S. limits on dark matter annihilation at the core of the Milky Way are the currently most stringent limits at TeV energies.W. Hofmann, July 2012